Solar Islands: A new concept for low-cost solar energy at very large scale
Posted by Francois Cellier on May 20, 2008 - 12:49pm in The Oil Drum: Europe
This is a guest post by Dr. Thomas Hinderling. Dr. Hinderling is the CEO of CSEM Centre Suisse d'Electronique et de Microtechnique SA. One of CSEM's most exciting projects concerns the design of a new class of large scale concentrating solar power systems, called Solar Islands 1. This article introduces the solar island design to the readers of TOD 2.
Introduction – the Problem
During the next decades, the generation of sustainable energy will become one of the main challenges of our civilization. Worldwide energy demand is expected to grow from about 10 GTep (1010 Tep [Ton Equivalent Petrol], or 5*1019 Joule) in the beginning of the century to 15-20 GTep by 2050. Some scenarios predict even levels as high as 40 GTep. An analysis of future global petrochemical consumption needs (i.e. energy needs and/or raw material for chemical industry) implies that early petrol shortages might already appear in the mid of the century.
The need for large scale renewable energy sources is underlined by the global warming due to increasing CO2 levels. CO2 is an unavoidable by-product of the energy generation process using any kind of fossil fuel.
Or, in simple terms: Not only are we running out of petrol, but the combustion of petrol causes major environmental problems. However, worldwide deployment of renewable energy in very significant quantities constitutes a huge effort of political and financial nature; incredible amounts of invested energy infrastructures are involved. In view of this, the start of this evolution is becoming even more urgent, already today.
While these facts start to be more and more accepted, there is still no global solution for renewable energy sources available. Such a solution should provide usable energy in very large quantities and at competitive costs, i.e. competitive in regard to today's energy prices. Current solar solutions are either not sufficiently scalable (they are only of regional nature), or they are too costly. In most cases, their underlying business model is based on massive public subsidies (which clearly is impossible at large scale deployment), or on massively increased energy prices (at least five times as high as today 3) which would simply disrupt the world economy.
Renewable Energy Sources
Among the many renewable energy sources, the potential of solar energy is at least one hundred times larger than any other renewable energy source (see figure 1).

Fig 1: Yearly global energy for different energy sources (logarithmic horizontal scale, source: Commissariat à l´Énergie Atomique, CEA, France)
Moreover, it can be shown that direct solar irradiation is the only source of energy that can satisfy the global long term energy demand – all other sources of energy are either too insignificant compared to the worldwide energy need (wind, CRW [combustible renewables and waste], tidal energy) or they are too costly (geothermal). Wind, CRW, tidal energy or geothermal energy can all be very interesting on a regional and local base, but they can by far not supply the necessary Gigateps of energy to satisfy the global demand. They have to be considered as auxiliary energy solutions.
Even nuclear fusion energy (should it become available soon, which is unlikely) cannot provide a global solution. Thousands of fusion plants would be needed in order to supply a significant amount of global energy; the ensuing technological and political complexity would be far too high.
Any solution for a future global energy supply must base on direct solar energy conversion at large quantities. Again, this does not mean that other forms of energy could not be interesting, but it means that a global solution can only be focusing on solar irradiation as the main supply of electric and combustible energy.
Classes of Solar Energy Converters
Today, there are four main classes of solar energy systems in operation or in development (aside from many other ideas in test):
- Photovoltaic panels:
Using the principles of photovoltaic conversion, solar light can directly be converted into electricity. This principle is extremely attractive, as the conversion into usable forms of energy is very simple; using little electronics, the output of solar PV panels can directly be fed into the electric grid. The problem with this solution is that the costs of such PV panels are still quite high, and the efficiency of the energy conversion is quite low (between 5 and 15%). Even though there is high hope that the costs will come down and that the efficiency will go up, PV solutions are based on semiconductor surface layers which are inherently expensive. It is doubtful that this technology will provide large scale solutions at sufficiently low costs. Another big and unsolved problem of photovoltaic solutions is the storage of the energy – a must for supply continuity over 24 hours a day. Future solar energy must be bulk energy, available according to daily fluctuating demands, not according to the position of the sun in the sky or the weather! Solar energy at large quantities implies the necessity for energy storage.
- Low temperature solar panels (collectors):
These panels use the direct irradiation of the sun to heat water. Temperatures of up to 100oC can be reached. This type of panel is highly efficient (heat is converted into heat). The heated water is relatively easily storable, even at large quantities. These panels are therefore ideal to generate a supply of warm water for domestic and industrial use. However, due to their low temperature, the conversion of the heat energy into other forms of energy (mechanical or electric energy) is very inefficient (see Annex 1).
- Thermo-solar high temperature panels and systems (100-350oC):
These systems, also called CSP systems (for "Concentrated Solar Power") collect heat energy from the sun (mainly visible and infrared irradiation), typically to generate saturated (but not super heated) vapor at high temperature (up to 350oC) and high pressure (up to 60 bars). The high temperatures are needed to increase the efficiency of the energy conversion from heat to usable electric energy. To get to these higher temperatures, the solar radiation needs to be concentrated, so that the surface-specific irradiation is corresponding not only to one sun, but to "many suns". Various examples of such concentrators have been developed, but there are two main types:
- Trough-shaped concentrators: The sun is focused to a centrally disposed tube, in which a liquid circulates that can absorb heat. Heat absorbents are typically water or mineral oils.
Fig 2: Trough Concentrator
- Extra Flat Concentrators: The same principle can be arranged in a lower cost arrangement, as in the following figures:
- Trough-shaped concentrators: The sun is focused to a centrally disposed tube, in which a liquid circulates that can absorb heat. Heat absorbents are typically water or mineral oils.
- Thermo-solar very high temperature panels and systems (>1000oC):
There are many examples of very high temperature solar concentrators. The high temperature allows for a very efficient energy conversion, but current solutions are still by far too expensive. It is hard to understand that they ever could become cost competitive.
Cost Considerations
The costs of a solar solution for very high energy quantities need to be similar to today's energy costs, or at least not much higher, as this would have a very negative impact to the world economy.
To estimate the maximal costs that could be accepted in a competitive market environment, we have to start by considering the solar irradiation which is defining the potential revenues. Near the equator it is in the order of 1 kW (kilowatt) per square meter at noon and clear sky. This corresponds to an irradiated mean power of ~250 W (as a mean over 24 hours, i.e. day and night) , equivalent to an irradiated energy of 6 kWh (kilowatt-hours) per day and per square meter. The efficiency of the conversion from solar energy to usable energy (delivered at user's site) is around 10%. In an optimal case (365 days of sunshine, latitudes 0-20o), this mean solar power corresponds to a converted energy of 220 kWh per year and per square meter. At a price of crude oil of around 0.07 US$ per kWh (if converted to equivalent electric energy), this translates to revenues of ~14 US$ per square meter and year. Assuming that 10% of the costs per square meter are used for financing costs and amortization (amortization time of 20 years) and that 10% are used for operational costs, the investment becomes profitable if the costs per square meter are below 70 US$ per square meter (see detailed calculation below).
All current solar solutions are at least 5 times, most often rather 10 times more costly than that. Therefore, new ways and new solutions are needed to provide solar energy at truly competitive costs, so that it becomes a commercially interesting issue, not just an idealistic dream.
It is unlikely that PV panels would ever be available at costs below 200 US$ per square meter (see figure 4). This is mainly due to the fact that the material of the PV panels (semiconductor material) is process- and energy intensive. Also, the advantage of PV solutions at small and medium scale turns into a disadvantage at very large scale. The wiring, power conversion and cleaning would be difficult, and, most of all, the storage of energy is difficult, too.

Fig 4: Photovoltaic module costs of various producers (US$ / square meter, without electronics!)
CSP systems are just the opposite: They are very expensive at small scale (the conversion of vapor to electricity is complex), but they are an excellent solution at very large scale. The reason is simply that at very large scale the active panel area becomes the main cost driver, while the auxiliary systems are much less important.
The conclusion is obvious: High temperature thermo solar panels (=CSP) are clearly the lowest-cost solution at very large scale, while PV systems are the best choice for small systems.
A New Solution
EFC (extra flat concentrators) panels, as described in figure 3, are already offering system solutions which are considerably less expensive than all other solutions. But they still suffer from two disadvantages which render them too costly. Firstly, as they need to follow the sun in its path across the sky, they have to make use of a precise and costly tracking mechanism. Secondly, they offer a large resistance to wind. They either have to be built very ruggedly (expensive), or they lose dramatically in efficiency (wind movement meaning de-focusing, therefore loss of efficiency). It does not seem to be possible at first glance to construct a panel which focuses horizontally, instead of vertically (i.e. turning left-right, instead of up-down), as the panels have to be linked to high pressure vapor lines that go from panel to panel:

Fig 5: Schematics of a line of solar panels with connecting high pressure tube
As the figure shows, it is not possible to turn the panels in this line individually; they can only be turned as a whole. This is where the idea of Solar Islands comes in: If one could put all panels on a very large platform and then turn the platform as a whole, the panels could be fixed horizontally on the platform (offering almost no wind resistance) and be focused by turning the whole platform, thus providing a very cost-efficient solution.
Floating Platforms
Based on the ideas of the author, CSEM has designed a very simple way to provide such a solution, i.e. a very large platform which can be turned as a whole. As the weight per square-meter by the loading of the solar panels is extremely regular (for instance, one panel every 10 meters), we can make use of a very simple principle: A large low-cost surface sheet (typically a plastic sheet) is fixed to a frame in an airtight manner. An over-pressure is applied below the membrane, thus exerting a vertical force. The amount of the over-pressure can be adapted to the specific weight per square meter. In this way, very considerable weights can be easily lifted (without lateral forces!). One tenth of an atmosphere of overpressure exerts a force equivalent to 100 g per square centimeter. This corresponds to 1 ton per square meter already! Therefore, it is easily possible to exert a sufficiently high overpressure to lift the membrane with the panels fixed on top of it.

Fig 6: The principle of "floating". Note: No lateral forces are occurring!
In addition to that, there are ways to turn the platform as a whole to focus the panels to the sun. CSEM proposes to do this in two possible ways:
- Solar Islands floating on water:
In this case, the easiest way to construct it is to design it as a spherical platform, which is formed by a swimming ring (typically made of pipeline tubing) over which the membrane is extended and which can be turned very accurately by means of hydrodynamic motors (spaced for instance every ten meters).
Fig 7: Principle of Solar Island
Fig 8: Turning with the sun
Fig 9: Focusing Principle
- Solar Islands on Ground:
Using a special construction on land, one can use exactly the same principle as described above, as shown in figure 10.
Fig 10: Solar Island on "terra firma"
In this case, a channel has to be built, filled with water or oil, in which the Solar Island can float just like on the sea. Please note that the length of the channel is proportional to the radius of the island, but the surface to the square of the radius. This means: The bigger the island, the less of a problem to build the channel – the costs become soon negligible in comparison to the total costs. The bigger the island, the more the costs are primarily defined by the costs of the solar panels.

Fig 11: Solar Islands – on high sea, near the coast or in the desert
This is why the concept of Solar Island clearly provides a lowest cost solution for solar energy, as the solar panels used are simply – glass! And the focusing mechanism is very simple.

Fig 12: Computer simulation of large Solar Island
Combined Systems
The efficiency of the high temperature solar conversion into electricity is estimated to be around 10%, as mentioned in the paragraphs above. This is a very conservative value; actual experiences let us hope to achieve efficiencies nearer to 15% than to 10%. In addition to that, there is the clear and interesting possibility to combine the energy conversion to electricity with water desalination and/or district chilling. Depending on the local situation of the site of a solar island, as long as it is either on land or not far from a coast, the co-use of the electricity generation process can be used to increase the overall efficiency considerably. Values of up to 18% seem feasible. At such efficiency levels, solar islands would generate electric energy in a commercially competitive way already now.
Energy and Profitability Considerations
Efficiency can be estimated in several ways. We know that it will be somewhere between 10% and 20%, from solar energy to electricity. Let us, for the time being, assume an efficiency of 15%. As outlined above, in very sunny regions at latitudes between 20o north and 20o south, we get about 220 kWh converted energy per square meter and per year, at a mean power of about 250 W solar power per square meter (clear sky). An island with a radius of 1.5 km, covered at 90% with solar panels, having an active surface of 6.4 square kilometers, provides a maximal power of 0.96 GW (Giga Watt) and a mean power (24h/24h) of 192 MW, generating energy of 1.5 TWh per year (1.5 billion kWh per year). This is corresponding to the energy of a small sized nuclear power plant!
Calculation Sheet: | ||
Radius | 1.5 | km |
Surface | 7'068'583 | Square Meter |
Conversion Efficiency | 15% | |
Active Surface Coefficient | 90% | |
Active Surface | 6'400'000 | Square Meter |
Cost per Square Meter (Panel) | 150 | USD |
Cost Overhead | 20% | |
Assumed Price per kWh (2008, no subsidies by Governments) | 0.17 | USD |
Mean Solar Power Per Square Meter (clear sky) | 200 | W |
Maximal Power Per Square Meter (perpendicular to sun) | 1'000 | W |
Sunny Days per Year | 330 | |
Mean Power | 192 | MW |
Maximal Power | 960 | MW |
Energy per Year | 1'500'000'000 | kWh |
Income per Year and per Square Meter | 40 | USD |
Income per Year | 255'000'000 | USD |
Cost | 1'152'000'000 | USD |
Operation and Maintenance Costs | 115'200'000 | USD |
Amortization and Interest | 92'160'000 | USD |
Profit | 47'640'000 | USD |
ROCE (return on capital employed) | 4.10% | |
Break-even Price of kWh | 0.15 | USD |
As this calculation shows, the business of Solar Islands starts to become profitable at an energy price of around 12 cents per kWh of electricity. The ROCE (return on capital employed) is already 5% at energy prices of 15 cents per kWh. Other important profitability parameters:
- Panel costs, estimated to be 100 US$ / m2, will be quite possibly lower at high quantities
- Efficiency levels, estimated at 10%, will be quite possibly higher, for two reasons:
- Maturity of technology will increase the performance
- Co-generation of cold (for district cooling) and sweet water production is increasing the overall efficiency of the process
We do firmly believe that a mixture of these three areas for potential profitability increases will allow for a very interesting business in solar energy at very high volume.
Photovoltaic and Solar Island
A special construction of concentrators allows combining the principle of Solar Island with photovoltaic panels (figure 13).

Fig 13: Photovoltaic panels combined with concentrating mirrors
As shown in this figure, the solar irradiation can be increased by up to a factor 10. This means that only 1/10th per sqm is photovoltaic material, 9/10th is of mirror material. Even at photovoltaic costs of up to 500 US$ per sqm, the mean cost per sqm is as low as 140 US$. This principle therefore not only allows for very cost-efficient PV solutions, but at the same time the fixation, cleaning and connection of the PV panels becomes much simpler. The concept of solar islands opens possibilities in CSP (concentrated solar power) and photovoltaic!
Cost Comparisons
The comparison of cost per kWh (i.e., not price – that is yet another issue!) is difficult to do. It has to base on a certain number of assumptions. Our estimations yield the following results (figure 14):

Fig 14: Cost comparison
The comparisons are made for latitudes of below 20o - at more northern sites the costs per kWh goes up in line with the reduced solar radiation (see Annex 1). It is obvious that all solar-island-type solutions are extremely cost competitive.
Next Steps
Many countries and many institutions have shown their interest for the ideas of Solar Island. CSEM has founded a company named Nolaris Inc., so as to be able to follow up on this interest. Nolaris is currently in a start-up mode, but it already pursues potential contracts in Malta, Qatar, Chile and Tunisia.
The most concrete partner is the government of Ras al Khaimah, the northern-most Emirate in the United Arab Emirates (UAE). Ras al Khaimah decided to finance a prototype solar island in the desert of the UAE near Ras al Khaimah City. The construction of this prototype island (diameter 100m) has started; it should begin to operate by mid of 2008. It will prove the feasibility of the concept and give a clear idea about the achievable costs.
References
[1] Patents claimed and deposited - all ownership of intellectual property rights are with CSEM S.A. and Nolaris S.A..
[2] Disclaimer: This is a conceptual description, meant to clarify new ideas for solar technology, so that they can be discussed and analyzed. Please do not definitely rely on this data, as it is subject to detailed technical discussion and feasibility.
[3] Solar electric energy is today about three to four times more expensive than electric energy from nuclear power plants. Solar "storable" energy (like liquid hydrogen or other energy carriers) needs to be generated from solar electricity (at least, based on today's technologies). The comparison of crude oil and "solar" hydrogen is therefore even less in favor of solar energy. Yet it is this form of energy (not electric energy) which forms the bulk of the global consumption.
Great article, with a promising outlook.
You mentioned that PV systems lack the ability to store engergy as needed, but I see little mention of how the CSP and solar islands overcome this problem. Does the fluid/vapor retain its heat all night generating power around the clock? One could assume the PV solar-island you mention would still have a storage problem to overcome.
Probably the most compelling thing for me with Solar energy on a large scale is that the best places to build them (near the equator - between the tropics) tend to be far away from the modern population centers that need the energy.
Transmission and storage will be as much (or more) of a challenge than building an affordable/profitable array.
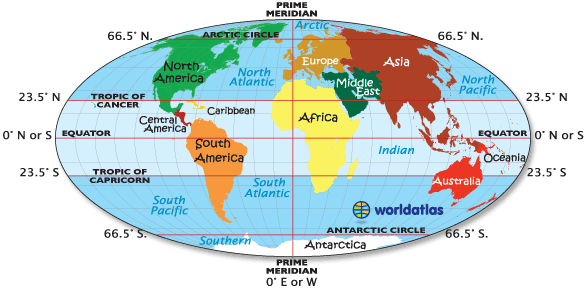
You can see this might work well for emerging energy users like India and China, but not so much for the US and Europe.
Guessing the islands will float just offshore and will be grid-connected by underwater cable. I'd be a little concerned about seaworthiness. Big waves could mess things up. Otherwise, very cool idea.
LoL Ya think?
Wonder what a tsunami would do to a floating "solar island"?
If they are out at sea, probably not a lot. Tsunamis are only a problem when they come to shore. Offshore the wave height may only be inches.
Yeah, but I was responding to Barrett808's post where he said that he guessed that the contraptions "will float just offshore." On the open ocean tsunamis wouldn't be much of a problem; regular ole wind-churned waves would be quite sufficient to wreck a "solar island."
I'd be much more concerned about hurricanes. For example, I don't think anything like this could be done off the Atlantic shoreline. The Pacific would probably be okay, though.
Kinda depends on where it is floating. If your are far enough out at sea, and we're not talking hundreds of miles you might barely notice a tsumani passing by that could easily devastate a coastal village when it makes landfall. However a cat five hurricane might be another matter entirely.
Having worked as a deep sea diver on oil rigs in the past and currently living in the greater Miami area I have a pretty healthy respect for the forces one might encounter out there on the great oceans of the world. Then again if you could sail these islands out of harms way given enough warning it might not be such a bad idea. I would love to see something like that kite surfing out in the ocean.
I wouldn't discount this for the US or Europe, as they both have solar CSP systems up and running now.
http://www.erec.org/renewableenergysources/csp-solar-power.html
"In Europe around 300 MW of solar thermal power plants are either operating or under construction. The installed capacity in Europe is expected to be of 500-1,000 MW by 2010 and an amount of more than 20,000 MW by 2020 is reasonable."
Yes. PV systems have a problem because they produce electricity directly, and electric energy cannot be stored easily/cheaply. CSP systems are thermal solar systems operating at high temperature values. They can easily store their pneumatic/thermal energy (high pressure/temperature) during the night. Of course you lose some of the stored energy, but the loss is economically and technically acceptable.
Another issue is the summer/winter issue. Solar systems provide more energy during the summer months, which is luckily when you also need more of it close to the equator (for cooling). When you build a solar power plant at a location farther away from the equator, e.g. here in Switzerland, you have a problem, because the solar system produces most of its energy during the summer, whereas we consume much more energy during the winter months (for heating).
So maybe you don't use it for power during the winter. You use the solar collectors for direct heat.
We need all technologies that we can get.
One of the beauties of small-scale solar solutions (collectors or photovoltaics) is that they are incremental systems. They may not provide the most efficient solutions economically, but economical considerations aren't the only considerations to be pondered.
A small-scale solar installation is something that every property owner with a bit of extra cash can do on his or her own, whenever it is most suitable. The technology is readily available; it doesn't take much of a planning; and it doesn't cost an arm and a leg. It should be possible to "convince" home owners to invest in such technologies either by suitable PR efforts or by legislation. Each installation on its own is a drop in the bucket, but together they can make a difference.
I'm also thinking that a universal power plant needs to be designed. Solar collectors to heat the working fluid, with alternate auxiliary fuel burners. No more pure fossil fuel burning plants.
Isnt's CRW and Wind a form of Solar energy?
We should not graph it separately, because they are interdependent. If we take some solar energy, we will diminish wind and CRW potential.
The most efficient form of use of solar energy was ommited. Photosynthesis uses 80% of incoming quantum of red light, leaving 20% as waste heat.
Unfortunately, the total photosynthesis efficiency in plants is much lower due to low CO2 and low Chlorophyl concentrations. But I believe, that these obstacles are technologically solvable.
The wind energy figure will have been taken at a relatively low altitude, perhaps 50 meters, and will have other limiting assumptions.
High altitude wind if practical could amply provide all the power society needs.
Likewise hot rock geothermal.
Likewise nuclear fission.
I don't have anything against this idea, but some of the argument is a bit tenditious - for instance, PV power may make up for it's higher cost by being close to the consumer, and not requiring a very low efficiency stage of production of hydrogen.
This sound useful in some hot desert areas, but too extravagant claims need handling with care.
Correct. The author assumed that the average wind turbine generates on average 20% of its maximum power, because it isn't constantly exposed to wind. Some wind turbines (in very good locations) may have efficiency factors that are three times as large, but on average, this is not the case.
Figure 14 was furthermore compiled for latitudes below 20o. At higher latitude values, wind energy becomes more profitable in comparison with solar energy, because at those latitudes, you experience stronger winds and less sun.
I think this concept has serious problems from the structural issues (requirements and dynamics) alone.
Could integrated sea power station be employed share the cost of one platform and infrastructure.
Could we have seaborne platforms, that:
- combine water colled (CSP)
- behind Photovoltaics PV,
- floating on island made of wave force generators,
- with submerged tidal turbines
- and with Wind Turbines mounted atop?
This could provide some energy at storm and during the night. Hopefuly we do not have still air with no waves at the middle of night during tidal peak at the same time.
Still, how can we transport energy to the shore? Can we dunk high voltage cable into the seawater, or do we need to build utility poles all the way to the shore?
How expensive is electricity transport from the sea?
In addition to Engineer-Poet's points the membranes dimensions will change significantly due to thermal expansion and contraction over the course of a day. I don't see how a flat (as opposed to cantinary curved) membrane can be maintained. This problem, as with many of the others, becomes more significant as membrane diameter is increased.
There are other issues as well, but this bunch need to be answered first I think...
Engineer-Poet -
We appear to be thinking along the same lines regarding structural concerns.
The first thing that struck me when looking at some of the conceptual sketches is that it shows the membrane responding in a perfectly vertical manner when subjected to overpressure from below. Unfortunately, that's not the way things work. When you subject a flexible membrane to a pressure differential, it will tend to assume a spherical shape (picture soap bubbles or balloons). So you have that problem for starters.
And if you make this thing big enough, you will indeed get wind-induced waves rippling across its surface, much like a wheat field in the wind, and this would surely throw the focusing out of alignment.
As I see it, putting one of these solar island out on the sea is totally impractical, because in addition to the problem of wave motion distorting the surface you have the little problem of it being ripped to shreds at the first big storm.
A problem, though not an insurmountable one, is that of moving the steam from the island to the power plant (assuming you're not going to build a power plant on the floating island). Perhaps some sort of steam-tight gland at the center might work.
Having raised those concerns, I will say that in principle, I kind of like the idea of having fixed flat collectors on a large rotating 'island'. I think a more practical embodiment of this general concept might be to make these units much smaller but to have the collectors mounted on a lightweight solid structure that would rotate about its axis on a series of concentric rails. I could picture these to be about the size of some of these rotating irrigation systems that slowly turn on wheels.
This is incorrect. The bridge (membrane) would hang through (sag) without overpressurization due to its own weight. The surpressure applied is calculated to just compensate for this force, thereby removing all of the lateral forces.
Francois Cellier -
I'm not saying that one would be unable to compensate for this natural tendency for even a mildly inflated membrane to bulge (just watch a large balloon during the early stages of inflation), but it is something that will have to be taken carefully into consideration. Maybe you will be able to balance it out by careful weight distribution, but I think it will be very difficult.
The fundamental problem that I see, based on my understanding of the concept as explained, is going to be the difficulty in maintaining a sufficiently flat and dimensionally stable surface so as to ensure that all of the collectors are focused on the receiver all of the time. As it is critical to maintain the desired angle of the collectors within a fairly narrow band, any rippling, distortion, or movement of the inflated surface is going to reduce the amount of solar energy actually transferred to the receiver.
It is hard enough getting a large array of collectors all perfectly aligned even when they are fixed to a rigid support, but attempting to do this on a 'floating' membrane subject to movement strikes me as quite daunting.
duplicate
I mention this down thread but it is worth making this explicit: In the azimuthal direction of the Sun, ripples don't matter. This is clear from the design since they would only translate to an effective change in altitude of the Sun. In the direction perpendicular to the azimuthal direction of the Sun, the only ripples that matter are those that have half-wavelengths comparable to or smaller than about three times the width of a collector strip. If the collector tube it tilted at the same angle as its reflectors in this direction wrt. vertical, then the sunlight remains focused on the collector tube. You only get different tilt for the tube and the reflector if the ripple has a small wavelength. If the wavelength is large, everything tilts together. So long as you can damp the amplitude of small wavelength ripples, there should not be a big problem.
Chris
mdsolar -
Thanks for clarifying that point.
I would expect that ripples and other types of surface distortions could and would take place at just about any orientation about the axis of this thing, depending on direction, strength, and variability of the wind acting upon the surface.
You indicated that as long as the ripples have a small wavelength there shouldn't be much of a problem. Well, what sort of minimum wavelength would you consider acceptable?
Again, it's not just the transients that I would be worried about, but mainly the basic problem of trying to maintain a flat surface on a huge flexible membrane. Nor would I discount the problems that would be caused by heavy rainfall, or worse yet, snow. But as these things would appear more suited for dry, sunny locations, such as Arizona or the Middle East, that may not be an insurmountable problem.
Actually it is the other way around. If the ripples have large wavelengths then they don't matter. They leave the reflector assemblies locally flat (though tilted). The compensation is not perfect. If you tilt the refelctor the angle of the reflected light increases by twice the tilt angle while the absorber only tilts by the tilt angle, but the aborber does tend to follow the direction of the deflection of the light. So long as the aborber is wide enough to handle the amount of expected tilt and still capture the light then there is no loss. It is really only ripples in the perpendicular direction (or the perpendicular projections of arbirarily oriented ripples) that are important because the parallel ripples just slide the reflection along the absorber.
So, local flatness in one direction together with not too large a tilt should keep the light focused.
Chris
mdsolar -
I know this thread is already a few days old, and I don't want to belabor the point (or at least not too much), but it still seems to me that even small changes in reflector angle will have an adverse effect.
For example, if a particular reflector is set at an angle of say 25 degrees and if the target receiver is say 40 ft away, then even a 1-degree relative change in angle will cause the center of the light beam to move about 8 inches (40 ft x sin 1 degree) either up or down. So, if the receiver is say a 6-inch diameter pipe, you will have moved the center of the light beam right off the receiver. And that is for a relative angular displacement of only one degree.
So what is it that I'm misunderstanding or not seeing about this?
These are very good questions indeed that deserve an answer, an answer that I cannot provide. I alerted the author to these questions, and I hope that he'll be able to provide satisfactory answers to at least some of them.
What Engineer-Poet demonstrated clearly is that size matters. Not all technologies scale up equally well, and the scaling problem needs to be looked at very carefully.
I am also inclined to believe that the "terra firma" (desert) solution may be simpler to work out than the ocean construction. I am also concerned that any technical problems with the platform will take the entire power plant off-line until the problem has been fixed.
A possible solution might be to build a ring of 20-30 individual Solar Islands of 100 m diameter each around a single thermal power plant built in the center.
In this way, if there is a technical problem with one of the platforms, the others will continue to produce steam (high pressure) for the turbine.
Also, grading the terrain would become much less of a problem, because not all of the individual Solar Islands need to be at exactly the same altitude.
The prototype that is currently being constructed in Ras al Khaimah has a diameter of close to 100 m. We'll know soon enough whether all of the potential engineering glitches have been worked out in its design.
If the results are positive, more money will be made available to replicate the design either on its current or on a somewhat enlarged scale.
It is perfectly feasible to raise the center part of the platform a bit so that water can flow off on its own. The angle of the mirrors can be calculated to compensate for the difference in height. Alternatively, you can adjust the location of the pipe to the focal point. The pipe carrying the liquid (water or mineral oil) to be heated doesn't have to be on a straight line throughout the platform.
You need a minimum pitch, or a random wind- or flow-generated variation will create a local low spot and water will immediately flow into it from all directions.
Your best bet is probably to forget the idea of a flat mounting surface. Forget the idea of rotating a mile-wide collector system too. Cylindrical inflated reflectors aiming onto pipes have none of the wind-oscillation or water-handling difficulties of a large flat membrane, and only have to be rotated on one axis. IIRC Daniels describes this experiment being done in the 1950's in Egypt. We can use fast, cheap and easy; maybe it's time to dust that one off.
It is not a question of one or the other. We need everything that we can get ... and this includes the best (i.e., most economical) designs for CSP systems.
At the current time, there are several CSP projects being under construction (or recently completed) as prototypes, i.e., primarily for experimentation, but simultaneously also for usage.
Some of them are cylindric/parabolic designs (similar to the Solar Islands, but with tracking devices associated with the individual mirrors). One such plant that I am very familiar with is the CESA-1 1 MW plant being built in Almería, Spain. I sat on the committee of a Ph.D. student who wrote his dissertation on creating a mathematical model of that plant.
Another design is the Solar Tower design, whereby sunlight is being focused on a single point rather than a line. This leads to higher temperature values, and thereby to a higher efficiency of the Carnot cycle (conversion of heat to work). An 11 MW plant of this type that I am fairly familiar with is the solar tower constructed recently outside Sevilla.
Solar Islands are a third contender that already proved itself sufficiently well to attract several million Dollars of funding for a prototype that is currently being built in Ras al Khaimah. As not much had been published on that third design in the past, I invited Dr. Hinderling to present his design to the readers of TOD, thereby placing it prominently in the open literature.
Which of these designs will ultimately win out remains to be seen, but until this judgment can be made, we should welcome this and any other potentially promising new designs with an open mind and with enthusiasm.
(emphasis added) Which implies "workable" and "not too expensive". I question whether solar islands fall in this class. What works in and around the sheltered Persian Gulf is going to face a very different environment in open ocean, or even the Great Lakes.
Remember who you're talking to, here. ;)
dS = dQ/Tabs
First off - amazing read, thanks.
I have several question (in addition the issues EP raised above):
- How do you plan to take out the heated fluid from the CSP collectors to the thermal storage and power generating equipment? I don't think this will be a trivial task for a moving platform like this... you either have to build expensive super insulated piping mechanism (and for a 0.92GW peak this should be some heat flow!) or you should place all of this under the membrane center - which is obviously the only option for sea-based units
- Sea based the membrane should have some compensating mechanism for sea currents and winds, or it will sail away... anchoring it will be challenging under the immense forces it will experience
Couple of ideas:
1) If wind is such a big issue with conventional CSP, wouldn't building wind shades be cost effective? Putting the thing under a glasshouse may look weird at first glance, but maybe it would work
2) Wouldn't building many small platforms (e.g. 100m in diameter) avoid many of the issues me and EP raised? A very small platform (10m?) may not even need to be built with a pressurized membrane, channels and such... just put it on a spinning wheel (though I'm almost certain this design has already been considered).
And one small criticism - 192MW should be the output of a very very small nuke... the standard size now is 1GW and up, which would translate to an output of 900MW and up on average. The fact we need 5 enormous platforms, 3km each to equate this just shows the extent this task is challenging. You don't need these little fact decorations, to make your points... I do believe that CSP has a bright future even without them.
But overall - amazing read, thanks again
An excellent post. I agree completely that CSP deserves the great majority of attention and funding. As you point out, it is superior to alternatives by orders of magnitude (but I think 3).
You are being too kind in assigning $0.07/kWh to oil. The relative efficiencies with which the energy carriers are used must be taken into account, and electrical transport with regeneration is ~2.5x more efficient than diesel. Money cost is also a slippery measure of feasibility, and maybe we should instead ask what portion of the workforce must be dedicated to energy production in the competing scenarios. My guess, this could be up to 40%, assuming the energy produced continues to support a modern industrial economy, so overall maybe $0.50/kWh is the limit.
As I understand it, your concept still requires single-axis tracking provided by the Fresnel slats, and I am skeptical thinking of all these mechanical linkages so close to seawater. Given a choice of having to contend with salt corrosion and dust, I would choose dust!
When I was living in Tucson a few years back, $0.07/kWh was approximately what I have been paying for electricity. Here in Switzerland, electricity is quite a bit more expensive. In most places, they sell "regular" electricity these days for $0.17/kWh. Regular electricity is electricity of the grid used for illumination and cooking. If you install a heat pump at your house (as we have - with geothermal support on the primary side and solar thermal support on the secondary side), the electricity company installs a second electric counter and sells the electricity used by the heat pump to you at a discounted rate of $0.12/kWh. I assume this is a government subsidy made available to push the technology onto the market.
Thus, different geographic locations will bear different prices. The $0.07/kWh may be the acceptable price for the location of the prototype in Ras al Khaimah.
Also, you need to distinguish between price and cost. Fossil fuel based electricity is sold currently for approximately $0.10/kWh on world average (here in Switzerland, we produce less than 2% of our electricity from fossil fuels - roughly 2/3 are produced from hydroelectric power, and the remaining 1/3 is produced from nuclear power stations). Yet, the oil companies reap a hefty profit, i.e., the production cost is considerably below $0.10/kWh.
Electricity produced from alternate energy sources cannot yet compete with electricity produced from fossil fuels economically. However, it may nevertheless be marketable by accepting a lower profit margin and/or in some cases a government subsidy.
Francois
You need to be a little careful in the quoting of prices. There is a substantial difference between the wholesale price that electricity generators are paid and the retail price you and I pay at our homes.
As a typical example the generators in australia are currently paid a market price for electricity of about US$ 0.045 per kWhr. The retail price we pay domestically is around US$ 0.13 per kWhr.
Any large scale generation scheme needs to compete with the wholesale price. Overlayed over this is the price you attribute to carbon. The current thinking has this as somewhere between US$ 0.05 and US$ 0.10. A renewable power generator therefore needs to be able to produce at around US$ 0.12 to be profitable once carbon trading becomes universally accepted.
While the solar island idea is a novel approach to the orientation of large arrays the viability will still come down to the cost of the installation. Given that the idea will still require "fixed" frames mounted on the membrane in order to train each mirror onto the collector i am not sure that you will save all that much over the costs for the Ausra linear fresnel reflectors.
A very interesting article. I do not say there isn't merit to the ideas you present, but there is a flaw that is common to all the technology "fixes" that hold out promise of our being able to avoid the radical retrenchment we otherwise face. All other resources are moving toward short supply, not just oil. Therefore costs will exceed anything you might project.
The virtuous cycle that blessed our ascension into the oil age, more energy leading to more technology and infrastructure, and these in turn leading back to more energy, now becomes a pernicious self-reinforcing downward spiral: less energy means less other resources, means less infrastructure, means less other resources means, means less other energy, etc.
In the end, we must face up to radical retrenchment towards sustainability. I don't say there's no role for solar on the way down. But nothing can save us from going down, IMO. This really is the new debate. Can the party continue by other means? Or do we face radical retrenchment? The debate about peak is essentially over.
Isn't that what a move to solar is? I note the parade of daunting problems that has already been raised against this particular embodiment, but my bet would be on some sort of CSP being an important feature of the future.
In contrast to solutions one can grow at home, big highly-centralised versions like this would raise questions about the cost/availability of what will soon be considered long-distance transport. In the world of inventing, nothing quite compares with actually doing it rather than dreaming it, even though the latter is a worthy necessary prelude to the former.
In terms of energy yes, but in terms of materials maybe not. This was the point I think DaveByGolly was trying to make. You seem to have overlooked this.
I tried to make a similar point on another post (it upset Pitt_The_Elder however) that a 20 MW gas tubine uses six tons of construction material (mostly metal), to generate this using wind would require more than 6000 tonnes of steel, sixty blades weighing 15 tonnes each plus concrete for the 20 foundations. Pitt replied and said a 6 tonne gas turbine uses 900 tonnes of gas per year. This was to miss my point, because today as I write this, that 900 tonnes is available because our infrastructure is in place to make it available, even if soon it may not be.
Equilibrium (approximately) of our current economic system is based on cheap high density energy, we are moving to a much lower energy density economy and materials, particularly metals are going up in price and the fact we are going to need to much larger "prime movers" or "energy gathering" systems in the future will only exacerbate this.
We developed our fossil fuel economy over 200 years or so at a pace where supplies of all materials could track demand at an affordable price. We now have the demand as before, but supply may be faltering, and material prices are rising. If the answer was obvious we would be solving the problem not discussing it.
Sorry to be a doomer, but a pessimist is an optimist who is aware of the facts!
Indeed but you aren't pooping enough! Not only do the "materials" (incl gas) have to be taken into account but also the inputs of transporting and travelling around to supervise them. For instance some solar construction in remote desert will need any number of tech experts jetting over there to do and monitor it, then accommodation and logistics for those visits, etc.
RPC,
The only reason I don't include gas in my example is because it effectively unlimited at the moment (or has been in the past at least), once you start to use gas to build a source to replace it it then you must include it, which is what I think you are saying (It is analogous to the logistic equation discussed recently on here I think). I'm not quite sure of your DOOM rating though! So
What is your doom rating? "0" being 100% optimist "10" being 100% pessimist
To read a bit about my (lack of a) doom-rating just click on my name above.
You have taken me too seriously, it was only a light hearted banter. I could not care less what your doom rating is!
they'll have the money because they'll have income from providing power. if not they can always just used computers to analyze the situation from afar. of course you also didn't factor in improved MPG of the fleet and airlines that will allow us to travel further.
This is indeed the invisible elephant in the living room.
The author writes in his introduction that we will see a reduction of availability of fossil fuels by mid century. He seems to buy the argument of BP that we still have 40 years of oil available at current consumption. He seems to assume that this means that we'll have affordable oil available for another 40 years, after which time we'll see a slow decline.
He writes that "as it takes a long time to develop new technology, we need to start this new technology already now."
Unfortunately, the problem is quite a bit more urgent than that. Peak Oil is now, and we'll see a reduction in the amount of available energy within the next few years.
Building HUGE new energy systems costs HUGE amounts of money, but even more importantly, consumes HUGE amounts of energy ... energy that we won't have available any longer.
If you are hungry now and you have one potato left, what are you going to do? Are you going to eat your last potato now, or are you going to bury it in the ground, knowing that it will produce 50 new potatoes next year?
William Catton, at the beginning of his book Overshoot tells the story of famine that was taking place in Russia. A visitor to a small village saw several villagers standing guard with guns over store of grain. He asked an old emaciated old man why the villagers did not eat the grain to relieve their hunger. The old man replied "We do not steal from the future". If we could form a similar social resolve in the face of an energy crisis, we might find a way to invest in new infrastructure even in the midst of a relative economic disaster. However, if we all insist on hanging on to as much of our current private wealth as we can for as long as possible we will be paralyzed, and no effective action will be possible.
RK,
The point is they needed a gun. This was probably required to protect the grain from folk that have access to no grain at all. If one believes humans will behave rationally for the collective good of all then there is reason for optimism, if, as I doubt, they won't then your latter point is the more likely outcome.
God bless the vodka!
You interpret the anecdote more negatively than I do. The old man did not say "I am not eating the grain because those bastards up their are preventing me". The village probably made a collective decision and posted the guards in the event that not absolutely eveyone was willing to do the right thing. In the same way if we made a collective decision to sacrifice current wealth for future wealth, the fact that some people would disagree with the choice would not necessarily doom our efforts to failure. I am not saying that I am optimistic that events will play out in this way, but I do not think we need to assume uniform sainthood in order for intelligence to prevail.
I was thinking more of people from outside the village, not the old man. I see your point about the villagers' common interest and think thats ok. In times of crisis humans do come together to defend common interests even people who previously didn't like each other. But I'm not sure at what scale it holds together, eg family, village, country or planet.
The other problem is people don't seem to anticipate a crisis very well. I will use a crude (and light hearted) analogy.
Give a person a glass of "cyanide" solution and ask them to drink it. Assuming they know its a poison that shuts down the matabolic process imediately and kills you at a similar rate, they won't drink it.
Now offer them a large glass of water laced with ethanol, also a poison (or at least the liver turns it into ethanal, which is). They will drink it and have a merry time and a few liver cells die, no problem plenty more to kill off. Keep going, this person will keep drinking, thinking he always has another day to stop before his liver packs in. Until one day it does.
The first glass of ethanol lead to his eventual death as much as the last! He may as well have drunk the cyanide and got it over with. Its perception of risk I think.
The majority of people who drink do not die of alcoholism. There is evidence that moderate drinking can reduce the risk of heart disease.
There's also no evidence that cyanide is fun.
There's hope for me yet then!
Private finance of capital only works well if such investments increase the total wealth of society. If we need to invest just to stand still or to prevent wealth from decaying away, then the prerequisite of giving a cut of the profits to the financiers will prevent vital wealth preserving investments from being carried out.
first of all, peak oil is not peak energy. secondly, so what if they use huge amounts of energy? they'll PRODUCE MORE energy. the energy will come from conservation and other reductions of demand. solar and wind right now are booming. in fact, solar and wind NEED scarce energy to become viable. quite the conundrum, no?
Sure. Just like in the case of my "last potato." They'll produce more energy, but WHEN?
The faster you have to switch to a new technology, the bigger the strain on the general economy. It may be too expensive for me to buy a new car right now and pay cash, but if the dealer allows me to pay off my new car over a period of three years in small installments, maybe I can afford it.
If the new Solar Island technology can be worked out in an economically sound fashion, humanity can easily afford to build 10 or even 100 of these islands on fairly short notice ... but not 10,000 of them, and that is how much it will take to only replace the oil. It will take three times more to replace gas and coal as well.
To replace the energy from all fossil fuels by Solar Islands at the current efficiency of 15%, we would need to cover 750,000 square kilometers by CSP mirrors, about 18 times the surface of Switzerland.
If we had enough time, we could stretch the investment out over a longer time period, but unfortunately, time is running out, or maybe, has already run out, and we simply don't know it yet.
Yet, I am all in favor of doing anything that we can ... everything, in fact. The question is not, which is the best technology. We'll need all of them simultaneously to soften the impact of the looming power down.
Perhaps we can convert some of those SUV's and reclaim all the steel and other matals. Using Solar furnaces naturally (once we have figured out how to make THEM!
This was the point I think DaveByGolly was trying to make.
Correct.
Wasn't that also true of wood, and didn't we use wood to build the machinery which let us use coal?
If materials are an issue, thin-film or String Ribbon™ silicon photovoltaic are possible responses.
We're going to have quite a bit of raw material available for some time; at 2 tons/ac yield, the USA produces the better part of a half-ton per capita per year of corn stover alone. Suppose you can turn this into 1 lb/ft³ insulating foam at 50% mass-efficiency. The share of a family of 4 could get 2000 ft² of foot-thick insulation, every year, from corn byproducts alone. If we ever reach the "Billion Ton vision" that will be more like 3 tons/capita/year of biomass. If we use it wisely (ie. only when electricity will not do), we can satisfy our needs.
Yes and we didn't move from the stoneage because we ran out of stone. This sort of nonesence does no one any favours.
We are still using wood and depleting the rain forests at an alarming rate
We are using more coal than ever before
We are using more oil than ever before
We are using more natural gas than ever before
I don't know how the curve would look if you plot the sum of consumption of the above materials against time, but I would guess we have used more in the last 30-40 years than any time before. each time we have found a greater more dense form of energy that previously, and more importantly at lower cost.
The key word here is "needs". Our current economic system needs constant growth in the total voume of economic exchanges to function in a "healthy" manner. Unless this "need" is eliminated not all of the clever engineering in the world will prevent the advent of social chaos.
where did you read this and please prove it. if worst comes to worst we will divert money(meaning steel and copper and etc.) that would go into building millions of cars and other consumer goods into CSP, windmills and etc. higher prices should also bring no more supply and more recycling.
I liken alternatives to the building of the transcontinental railroad. they build the railroad in the middle of nowhere and it was tough to get supplies on roads. so how did they do it? well the gutted it out until they built enough track and infrastructure so that the track they build was used to bring up supplies to build more track. genius! we'll do the same thing. we'll grit out teeth(like higher prices are causing now) and renewables will be the track that helps us build new renewables which will dig us out of the hole.
if you are a doomer you are saying the way we use oil now is the most efficient and not being able to use oil the way we do will cause disaster. this flies directly in the face of the message we have gotten from people like Kunstler, Heinberg and TOD- that we waste oil. 40% of our oil use is to simply get people to work in one car with one person in it incredibly wasteful!
so there is only one view you can hold if you're a doomer and that is we use oil incredibly efficient and changing our ways will mean disaster. obviously this is not true.
John
You can't prove materials are moving towards short supply any more than we can prove oil is. Its just highly probable due to limited processing facilities and the fact mines for certain metals (Tin in Cornwall and gold in Wales) are reopening after shutting years previously. Its equally foolish to assume we will always find the resources we want at the rate we want no matter how much we want. There is also talk of reopening some of the uk coal mines that previously had no future. Not proof, but some degree of evidence that shortages are on the way.
We actually waste all oil (and energy) because we used to live without it and probably will contiue to live when its gone but not with the lifestyle we have today. How we waste is purely subjective depending on what your priorities are and who you are prepared to sacrifice. JHK and RH are two of the biggest doomers I have come across and neither believe we are going to pass this period of human history without much pain. I suggest you read "The Long Emergency" and "The Party's Over", or if you have, read them again because you have obvoiusly got the wrong end of the stick of the message these two guys are giving. If this fails to convince you, listen to Heinburg and Kunstler et al in the film "The End Of Suburbia"
that just proves we're in a commodity bull market.
I've read alot of Kunstler's work and some of Heinberg's. I've seen the end of suburbia and have had to deprogram myself from that type of linear doomer thinking.
An investment in wind turbines today will yield a fairly constant average yield of energy for the next 20 years or so. Today's investment in PV is warranted for 20-25 years and may generate usefully for upwards of 50; the cost of PV is dropping rapidly and the EROI is on a steep UPWARD curve. Where's that downward spiral?
I won't argue with "radical", but "retrenchment"? I don't think so. There's 1200 GW of wind power available in the continental US, and another 900 GW potential on the continental shelves. This is 63 quads/year of pure electricity, while the USA currently uses just 105 quads/year of energy from all sources. Electricity goes a lot farther than lignite; we'd do just fine on wind alone, and increased efficiency and solar would allow a considerable increase in our standard of living.
The downward spiral is in the absolute values, not in the percentages of change. Oil may decline by 3% annually, while solar is increasing by 10-20%, yet oil will still win out, because currently, we are receiving close to 30% of our entire energy from oil, whereas we are receiving considerably less than 1% from solar.
Furthermore, time is working against us. As we move further down the rear end of the Hubbert curve, the annual percentage of decline in oil will increase, whereas the annual growth of solar will decrease, as solar conquers a larger share of the market.
I am afraid it's already too late to avoid the power crunch altogether, but this doesn't mean that we should give up and go down without a good fight.
I agree that, in the long run, we may be able to play Münchhausen and get ourselves out of the swamp by pulling on our own hair (bootstrapping technologies), but before this happens, a number of hard years are waiting for us ... and for our children who have never seen anything alike.
Small things can have truly radical rates of increase. The US increased its generation from solar by a factor of ~25 between 1988 and 1989. Installed wind generation in the US rose more than 50% in 2007. That's the production side. I suspect that official US figures are not counting grid-tied PV systems on private homes, because those keep going in at a good clip.
The USA could cut oil consumption by perhaps 15% with the simple expedient of substituting Ford Focuses for F-150's (even more with Priuses). Another 5% is available just by slowing down, and we could have it tomorrow. That takes care of several years of 3%/year declines, which we aren't seeing just yet. Vehicles like the Chevy Volt and Aptera can substitute other energy supplies for oil.
We have a lot of work to do to get ready for the real declines, and the will doesn't seem to be there. Yet. $5.00/gallon gasoline will create the will to get petroleum out of our lives, even if the public perception of the culprit is wrong.
My parents knew Depression and war from the time they were small. My children (who aren't born yet) will also know only the world they are born into, and see what their efforts help make it. Thus has it always been.
True. By far the most economic energy policy is to consider energy savings. If we could save faster than the decay of the Hubbert curve, then Peak Oil would be a no-event.
The best potential in energy savings is on the one hand in private home heating/cooling systems, and on the other hand in private transportation. Industry has already done a lot to optimize its energy costs for economic reasons; the private citizen has not yet done so.
I assume that they do account for a part of the grid-tied PV systems, but they don't account for stand-alone systems at all. The statistics can only account for energy that is being sold, i.e., passes through some kind of counting device. Energy sold to the grid from a grid-based PV system will be counted, whereas energy consumed locally without ever passing through an electricity counter will not be counted.
Interesting. This may be true (depending on how big a 'very large scale' is), but I question this conclusion. Even really small (i.e. one house) electrical generators are much cheaper than an equivalent photovoltaic system. As I understand it, a concentrating solar power station is just like a generator, it's just a heat engine. The only difference is that rather than getting its heat from burning gas or diesel, it gets it's heat from the sun. So even in a small scale concentrating solar power is probably better.
Of course, if the scale is small enough (like a calculator) then a photovoltaic system is a better choice. But for the purposes of electrical generation for houses concentrating solar power is a better solution.
This point was cover well enough in the article. If you are going to use CSP in your house you would need to invest much larger amount in expensive common overhead - piping, turbine, generator, installation etc. etc. You won't be able to add thermal storage - when it is small the thermal losses will be huge. You will not be able to achieve high efficiency because the system would be small and not able to achieve higher temperatures etc etc. And where do you plan to put it? You can't do that on the roof, and at 20W/m2 average output a system that generates just 1kW on average (what an US household usually uses) will take 50m2 of your backyard... In contrast the overhead of a PV system is very small - you've got just electronics and installation.
Thus a household CSP will cost you way much more than if you opted for PV. I would speculate it may cost you more than your house costs. But if those huge upfront costs were spread on a large enough installation the picture would look differently. I can't understand why so many people fail to grasp the concept of economies of scale easily.
[edited - it's 50m2, not 500]
I understand what economies of scale are. I also understand that a larger CSP system would be more economical than a smaller one. In addition I accept that for a really small system a photovoltaic system would be more economical. The question is: At what point does a CSP system become more economical than a photovoltaic system? That's an interesting question, and I have not seen a good answer for that question. You, and perhaps the article, claim that for a single house a photovoltaic system would be more economical. This is possible, but the question has not been definitely answered.
I am interested by one of the things you mention: You say that you wouldn't be able to add thermal storage for a small system because losses would be huge. I do not believe this to be so. Losses would be higher than for a bigger system, but they would still be acceptable. In addition a thermal storage system would be much cheaper than batteries for a photovoltaic system.
You mention that it would take up too much space. Well, if someone wants a solar power collector for their home, they have to live somewhere away from power lines. If they lived near power lines, it would be cheaper to just buy it from the grid. If someone lives somewhere so remote that there aren't any power lines available, 50 m2 of space isn't a problem.
What you fail to understand is that there is a 'sweet spot' for the size of a solar power operation. If a power station is too small it is uneconomical, but if a power station is too big it is also uneconomical. Everyone involved in concentrating solar power seems to be thinking big, bigger mirrors, bigger collectors, more power, but I'm not convinced that this is the right avenue to take.
Your question requires an expert analysis. Common sense dictates that the larger it is the more economical it would be until it hits some limiting factors. For conventional power, nuclear for example, the limiting factor is how large an equipment can be physically transported on site and also the transmission infrastructure.
For CSP I would expect it to be similar. You may have a point that medium size (community level?) CSP plants may make sense. But certainly not on household level, no way...
As for storage, the energy stored is proportional to the mass of the heat medium, which is proportional to the cube of the storage tank diameter (assuming spherical tank). Thermal losses however are proportional to the tank surface, which depends only on the square of the tank diameter. So a storage tank for a 10kW plant will lose energy 10 times faster than of a 10MW plant. A large plant can also afford for a better insulation etc.
I forgot to mention one critical thing about solar power of ANY kind.
Contrary to the assertion here, given current technology (even allowing for its improvement), solar power (PV, CSP or whatever) can not and will NEVER, EVER be the predominant source of energy for the humanity.
The reason can be summarized in just one word. The reason is called WINTER. Even in relatively temperate climates (20 to 30 degrees latitude), winter insulation is about half the summer insulation. Schemas relying on bringing power to Stockholm or Moscow from the equator, have the same probability of happening as those solar power satelites do. A more probable thing to hope for is the development of cheap, large scale electricity storage. But for this there are no more indications to be on the radar screen than zero-point energy or desktop fusion.
Possibilities for dealing with the winter/summer insolation difference are either to overbuild your solar capacity or to accept lower efficiency of use of energy intensive capital equipment. Both of these options will be costly, but not by more than the factor of the winter/summer insolation difference. If your goal is to reproduce the current standard of living or to go on continuously increasing it, as has been happening for the past two centuries, then such strategies will substantially raise the barrier for using solar energy to continue BAU. If on the other hand your goal is to maintain a decent quality of life with minimum consumption of resources and with minimal environmental impacts, then such a strategy might have signficant marginal utility compared to a return to neolithic technology.
Theoretically you can use some combination of those. Practically nothing like this will happen. Solar thermal is already costly enough even in it's simplest possible version, even in the best possible locations. Adding more complexity and even more inefficiencies and preconditions will make it prohibitive. How are you going to sell it to countries like India or Kenya? Or Canada and Sweden? (6 months a year with almost no energy, yes, thank you)
If it was the only energy source at hand, maybe our society would have somehow swallowed the pill and undertaken this vast enterprise - accepting substantially reduced standard of living along the way. But there are readily available energy sources, technically and economically vastly superior to that - coal, tar sands, nuclear power etc. etc. Even biofuels are easier. And there are no signs of depletion of any of those. All the talk about it is pure speculation for the hypothetical faraway future. Oil and NG are even more superior than those, and will continue to be so even decades after they have peaked. It simply won't happen, not because it can't happen but because we will choose not to do it.
What people dreaming for a "solar future" are actually pushing for, are trillions of dollars of public money spend on something which a free market would never provide. In a competitive world this will never happen, because those who will opt out will outcompete the rest.
Coal, tar sands, and biomass are not solutions for long term sustainable global economy economy at OECD levels of economic consumption. You can make a better argument for nuclear energy as a possible solution, but building enough nuclear reactors and an all electric infrastructure for transportation, construction, farming, etc for 7 billion plus people is also a 'vast enterprise'. I frankly think that we are going to have to accept lower material standards of living whether we like it or not. Certainly we cannot go on getting richer forever. I am not promoting solar energy as a miracle solution to our problems. I agree that in the long run solar energy will not be extensively used unless it makes economic sense. But I think that it is highly likely that many things that have made 'sense' during the era of cheap oil are going to stop making sense in the not too distant future.
"Coal, tar sands, and biomass are not solutions for long term sustainable global economy economy at OECD levels of economic consumption."
That's kind of strange. If they are not solutions how come that together with oil and NG they are already providing 87% of the world energy? While solar power is providing... hmmmm 0.1%? It's all about the money. An energy source won't be developed if it's costs are not competitive. Solar is not even close, and existing FFs are projected to last long enough. Actually they will be more competitive long after they've peaked.
So what do you plan to do? You can't tell an utility not to invest in a Coal Power Plant, because in 200 years the coal won't be there. Nobody plans that far. After all FFs are almost entirely depleted, after all uranium (after breeding and reprocessing) is depleted, after all land is depleted... maybe in hundreds of years we will get so desperate that solar thermal should get on the top. But then in 4bln. years the sun will die, so it turns out solar thermal is not a "long-term" solution either. Goodness
Let take these three sources once at a time.
Tar Sands: Only a small fraction of the worlds total hydrocarbon fuels come from tar sands. The oil geologists that I have read claim that this source will never provide more than a small fraction of today's total hydrocarbon usage. This in not a path for China, India, Afric, and South America to attain to OECD standards of living.
Biomass: The issue is how much more demands can we make on biomass without destructive consequences to food producition system and to the biosphere generally. From what I have read the answer is probably not that much more.
Coal: The 200 year claim with respect to the supply of coal is very much in dispute. Jean Laherrere has attemped to do Hubbert style analysis for coal and claims that a peak in the coal supply may be only a few decades away (We know how wrong he was about peak oil). For decades we have been taking the attitude that until the market sends definitive signals regarding the finite supply of oil we should go on burning the stuff like there is no tommorow. It is now evident that this was not an intelligent strategy. Why should we repeat the same stupidity with respect to coal?
I am not saying that we should start shutting down coal fired power plants or even that we should never build another new one. I am saying that we should start thinking intelligently about the future rather that trying to fill up our landfills with junk as fast as we possibly can and dumping pollution into to the air, soil and water at steadily increasing rates until cold hard necessity prevents us from doing otherwise.
where is it written in stone that they would? the EU has a high standard of living and uses about 40% less oil per capita than we do and they get a long fine.
Roger, if you are looking for a clean, endless, cheap, universal, reliable and if possible totally invisible energy source I suggest you look it in some alternative universe. In this universe we have to make do with what we have, and every one of the energy sources we have at hand has some issues - every single one of them!
Virtually all substitutes you defend based on some ill-defined "sustainability" criteria don't meet the technical and economic criteria - and these are absolutely necessary to even consider them as a "main horse". In the case of solar thermal - it is not even close to being competitive and reliable enough to meet a substantial portion of our everyday needs. It's also far from universal, as all Northern countries will be left freezing in the dark if they had to rely on it. This doesn't mean it doesn't have a place, only that it's place is limited.
If we are to "think intelligently" then we should encourage what is good for us and future generations and discourage what is not. But we can't plan for future generations too - this defies common sense! Beyond 30-40 years it's not planning it is guessing. For example we know for certain that the CO2 we release now is harming our environment. Some people including me defend nuclear as a CO2 free substitute. And then some "sustainability" experts jump and say "we shoudn't, we will run out of U in xx number of years". WTF is that? I will leave aside how unrealistic assumptions were in those xx years. Even if this were the real numbers, a solution that could last even 50 years is worth persuing, because even if its time comes to an end there will be time to develop the next available substitute. And there are always substitutes - in the end doing without is the substitute of last resort.
there are some very good points here.
I am not looking for miracle energy technologies and I am not rejecting nuclear power. I am rejecting an economic system which assumes that we are going to go on getting richer forever. Our economic goal should be to provide ourselves with a good quality of life with the minimum consumption of resources and the minimum environmental impact consistent with that quality. If this means 'doing without' 40 inch plasma screen televisons, 100 watt per channel stero systems, jet skis, muscle cars, 6000 square foot starter castles, jet airplane tourism, etc. then so be it. I certainly do not want to go backwards in terms of health care and sanitation, but the idea that our grandparents lives were a pychological hell because they did not have all of the consumer toys that we have, or because they could not jet around the world the way we do is nonsense. There is more in heaven and earth than is dreamt of in modern consumer culture.
I completely support you on that, but this problem is of our society which allows many people to spend their lives in aimless consumption while millions, actually billions of other huamn beings are starving, not having healthcare etc... It is a problem of our society, it's not a problem of what type of energy do we use. I fear that all things being equal oil and NG will be replaced with the dirtiest of fuels - coal, tar sands etc. based on pure economics. I don't expect PO to bring social justice, just the opposite - the poorest will suffer the most, while the richest will be the last to change.
Having said that, it is our duty to develop clean and low-cost solutions, suitable for developing countries, as well as developed ones. Solar thermal could be ideal solution for the developing South and I'm quite enthusiastic about its future there.
Here in Europe, there is currently a net flow of electricity from the North to the South, i.e., if we build more solar power stations in Spain and in Italy, we actually reduce the Southward flow of electricity and the losses associated with it.
Correct. I am all in favor of replacing aging nuclear power stations by new ones to grant us a few more years to switch over to a more sustainable technology. It's a proven technology, and it is needed in order to prevent that older nuclear power stations will be used beyond their safe lifespan once the oil starts to become either unavailable or at least unaffordable.
Yet, in order to replace fossil fuels by nuclear power stations, we would need to build 10,000 new nuclear reactors, and at least in PWR design, this is not possible, because we cannot produce uranium fast enough (same problem as with the oil). In order to make this work, we would need to switch to FBR (fast breeder reactor) designs that produce more fissile material than they consume, but this is also problematic, because FBR reactors generate weapon-grade plutonium as a byproduct.
Unfortunately, there is no good solution ... only many that offer small contributions and that, together, may possibly still be able to prevent the worst-case scenario.
I don't want to hijack this thread towards nuclear power. Just want to mention that if 10,000 nukes would look hard enough, 50,000 of these platforms with all their infrastructure etc. will look nearly impossible.
Which does not mean we won't or shouldn't persue either one of them... as you said - every little bit will help, and the concept you presented looks feasible.
As for the flow of electricity - I agree, but even Southern Europe doesn't have good enough solar resources and the seasonal fluctuations are quite bad. Europe also lacks enough free land, and the land of the south is mostly rugged. We may have to build them offshore, and this poses extra challenges. Overall I would support my taxes to be spent for a pilot project on that - I think it would be worthed.
Replacing US motor-fuel consumption with nuclear would take perhaps 180 GW of generation, give or take. This is about 115 of the latest 1.6 GW powerplants. It's hard to say what it would take to substitute for the rest of oil consumption, but in the case of industrial process heat it may be possible to supply much of it with the waste heat from those same nuclear plants. In the last resort, generate more electricity.
If I understand the details of the Molten Salt Reactor technology (corrections accepted), it would be feasible to run it on spent PWR fuel just by converting it to fluoride salts. That would also burn the plutonium. The neutron spectrum of a fluoride salt reactor is not hard enough to be an FBR, so that's proliferation-safe. Besides, if we're talking about the OECD we have nuclear weapons already; it's the rest of the world we have to worry about.
Sort of. What you're describing would be a liquid chloride fast neutron reactor with a very hard neutron spectrum (harder even than liquid metal fast breeder reactors.) Liquid fluoride thermal reactors could run on a start charge of enriched uranium and some plutoinium, but the solubility of transuranics in most desirable fluoride salts is too low to truely make them actinide incinerators. However, given that a LFTR only burns 1 ton of thorium per GW/year (compared to 200 tons of uranium per GW/year) and thorium is three times as abundant as uranium, the notion that fuel supply is limited this side of ten thousand years is silly. (Well, given how abundant even uranium is, fuel supply concerns even with light water reactors are silly, but thats another dead horse)
Liquid chloride reactors present proliferation problems. You can use the blanket of depleted uranium to make very high quality plutonium. They're desirable because they can incinerate any type of spent fuel, destroy long lived fission products (Tc-99 for example) and have the highest breeding ratio of any critical reactor designed, as well as being far safer than liquid metal cooled fast neutron reactors with more passive safety features. The breeding ratio of a liquid chloride reactor can be used to breed start charges of U-233 from thorium for liquid fluoride reactors. However they're also unnecissary, as mentioned earlier (even though from a technical standpoint U-233 is the most desirable thermal reactor fuel.)
Liquid fluoride reactors present far fewer proliferation problems, because most designs contaminate the U233 with U232 that has strong gammas in the decay chain, making nuclear weapon maintenance and handling prohibitively costly, as well as having a breeding ratio very close to 1, such that if you attempt to divert U233 for weaponization, your reactor will shut down, unless you feed it more enriched uranium in which case you have the facilities to make U235 weapons anyways.
Whats really silly is the notion that any country would reject nuclear power development because of proliferation concerns in favor of a lower standard of living.
I did say "fluoride", not "chloride". CANDU reactors can burn fuel with the reactivity of natural uranium, which includes spent PWR fuel. I assume graphite-moderated reactors can also. What's the obstacle with a liquid fluoride reactor?
Seems to me that it wouldn't have to be; even if you could only dissolve e.g. 1/5 of a spent core's inventory of plutonium in an initial fuel load, you'd be burning it off continuously and could keep adding more until it was all gone. The same would be true of U-238 from the spent PWR fuel; breeding and fissions are rare, but eventually it would all burn up.
I've been wondering why people seem to have been voting for that for years. Maybe because the true natures of the options were obscured by so much propaganda? Now that the fog is dissipating, public opinion seems to be aligning with the position I've held since my teens.
This means it's time to get out in front of the pack again. ;)
Here's hoping that we've got a stockpile of the elements to make Hastelloy-N.
They can, but they cant burn it all without excess fissile loading because of parasitic neutron loss. Theres other strategies for getting around this discussed at Kirks message board now if you like:
http://www.energyfromthorium.com/forum/
Well, they've never had to pay for it. There's allways been coal there to back it up.
My whole point was that if we make it predominant (say 50% of energy supply), how are we going to cope with the seasonal fluctuations? Direct solar power can not be stored, or has very limited options for storage.
If winter insulation is let's say half on average of summer insulation, how we are going to handle 25% reduction in total available energy? For comparison - oil represent about 40% of the total energy mix... the "winter effect" would be pretty much like halving the world oil supply. Of course we could compensate with other energy sources which can be stored more easily, but which ones can you think of? Can we really afford to build a whole new fleet of our coal/nuclear just to cover for solar in winter? And if we do that - why would we need to build solar at first place, when coal and nuclear would have been able to handle the whole year by themselves?
It simply doesn't make sense when you think about it. Direct solar is bound to remain a complementary source, just like wind, and that's all folks. A large scale storage may change that, but I wouldn't hold my breath on that.
Fluctuations in insulation are only significant at higher latitudes. Close to the equator there is very little seasonal variation.
At higher latitudes, CSP systems aren't effective anyway. At those latitudes, we may be better of using wind power.
Finally at lower latitudes, we need more power during the summer (for cooling), whereas at higher latitudes, we need more power during the winter (for heating). Grid solutions allow to compensate for some of the seasonal fluctuations by shipping power to where it is momentarily needed.
Virtually all developed countries and maybe 95% of the world energy consumption is in countries beyond 20 degrees latitude (north and south). Even in Sahara (sometimes proposed to power Europe if not the world) seasonal variations are very huge.
Claims that direct solar will provide the bulk of world energy imply one of the following very costly solutions:
1) A global electric grid to bring energy South to North - something Stuart Stanifort was suggesting a while back
2) A viable storage option, most likely hydrogen
I wouldn't bet on either of them. A global electric grid won't happen because of political considerations, aside from the technical ones. Will the global North agree to trade dependence to Middle East with Sub-Saharan Africa? I don't think so.
Hydrogen will be even more costly than the global electric grid, because we will have to double the initial investment in solar, just to compensate for the loss in efficiency, plus the pipelines to bring H to the markets, plus the conversion back to electricity... not realistic either.
I think solar energy will be used where it is produced. No paradigm shifts on global scale with tremendous price tags on them will ever be implemented.
That's funny, solar has been the ONLY source of energy for the history of the planet until we started buring wood and oil. The benefit of solar is that it covers 100% of the planet. To say that it can never supply the world's energy is being entirely short sighted. They are already developing paint based solar collectors and it is not unreasonable to believe that the technology could one day be in dyes that are in your clothes. The true benefit of solar is that the technology can become as small as chlorophyll and collectively can combine to produce large amounts of energy and be entirely inconspicuous. If you combine that with more efficiency in energy consuming objects then you can easily get 100% of our energy needs from solar.
The main problem that we need to solve is not producing enough energy but using ALL of the energy we produce.
We don't speak the same language here... by solar power I mean "direct solar energy", with the two major technologies to harness it - solar PV and solar thermal - and this is the common understanding of the term. FFs, hydro, biomass, wind etc. are forms of solar energy which are supreme to direct solar in that they are much more concentrated. And you misplace the argument - the challange has never been to "find enough energy". If you add oil shale or tar sands, or methane hydrates FFs would also be virtually limitless. The challenge is to harness it, and here is when solar faces some serious problems - just thing about it - 5 rotating platforms, 3 km in diameter - the size of a small city - would be needed to equate the output of just one nuclear power reactor. By no account an easy thing to do.
BTW nuclear ultimately comes from gravitational energy, so you are not entirely correct that all our energy comes from solar fusion.
Where in the world does this claim come from? Uranium and Thorium (If we ever start using it as fuel) come from fusion in the cores of super nova.
You are absolutely correct, but the fusion you are talking about is at an energy loss. The energy that went into forming those heavy atoms (all elements beyond Iron IIRC), came from the gravitational energy released during the rapid collapse of the super nova core.
Solar can never be the only source of energy, but it may be the predominant one.
The reason for that is that most people live in hot areas.
In those regions cooling is the predominant need, not heating, and for non-peak use solar fits the bill nicely - in much of the US, for instance.
Where reason is left behind and we enter the realms of fantasy is in seeking to use solar for base-load and for all areas, including northerly ones.
For those regions a combination of wind and nuclear has the right profile, and if used in reasonable combinations you immediately enter the realms of practical engineering, and knocks noughts off the end of the sums of money needed.
Molten salt thorium reactors which we have a fair idea of how to build within current engineering could provide power for many millions of years.
If the ideologues would get out of the way we have the means within our grasp to power society in both hot regions and cool.
C'mon, Dave, you're falling back to your old habit of broad pronouncements. Your objection is unduly harsh and dismissive while being inaccurate. The only constraint - assuming plants can be built of either PV or ST - is transmission. There is tons of space in very sunny areas to build massive solar plants. The only constraint would be as mentioned above. I would posit there really is no constraint conceptually: transmission losses can be calculated; you simply build enough plant(s) to satisfy all need.
Done.
To say this is fantasy is absolutely wrong. Impractical? Maybe. Expensive? Maybe. Fantasy? Bull.
Cheers
I can see where you are coming from, and on a theoretical basis one can generate energy in loads of ways - for instance by ocean heat gradients.
The thing is though that we have a problem now, and to move towards solving it we need something that we can do right now, and it has to be reasonably cheap as money post peak is going to be tight.
I mention molten salt thorium reactors just to show that we don't have to run out of fuel for reactors, but we have the technology right now to make a major build of conventional reactors.
Building CSP in desert areas would actually be much more practical in the US, as it would be under the same sovereign power and both the transmission lines and security of supply would be easier.
On those lines the recent Scientific American article is perhaps as good as it gets in concrete proposals.
However, it started to fall apart when looked at in detail, basically because trying to force solar into doing things it is not good at is expensive.
Problems include storing the energy, which is untested on a large scale - their solution involved compressed air, and used vast quantities of natural gas to re-heat it.
Solar thermal schemes for Algeria use co-generation, and are basically a natural gas plant with a bit of solar thermal to help out.
Schemes in Spain are in the 50MW range - and that is before allowing for solar intermittency, so they are equivalent to around a 10MW fossil fuel plant.
You would also need to solve the non-trivial problem of providing cooling water.
Critics of nuclear power point to the difficulty of providing water for cooling when there is a drought, well, as they are located in desert regions CSP has that in spades.
Dry cooling is both expensive and reduces output.
Can all these difficulties be overcome?
Yes, technically and on a small scale at great cost.
Can they provide a solution now to societies energy needs at any affordable cost?
No way, they are an expensive distraction, and not within current engineering.
As a solution to energy needs in northerly areas I stand by my statement that they are fantastic.
As a contribution and used appropriately in suitable areas they will provide a terrific resource.
It's all about using the right tool for the job, and in northern Europe the right tool is nuclear energy we have some fifty years experience of how to do that.
It is folly to throw away such a major resource and rely on technologies used where they are least suitable, and where we have no experience of utilising them on remotely the right scale.
FWIW the most suitable renewables for large scale use in northern Europe would probably be deep sea wind turbines, where most of the energy comes in winter, or hot dry rock geothermal, which might someday be able to supplement nuclear for base-load.
It is very early days for both in their respective technologies, and both need much further development.
This looks like a pretty good idea to me - but let's wait until some are built commercially before we all celebrate.
A few questions:
1) This still doesn't solve the problem of power at night, so you'd need to convert the energy to hydrogen.
2) If you convert the energy to hydrogen you lose about 40% of it's energy.
3) With this loss of energy, is there enough economically viable land to provide the power we need?
I could envision many islands like this floating in the sea and producing hydrogen. Special tankers could visit them and "offload" the hydrogen. Probably it could be done, but given the various inefficiencies and all those extra costs, I doubt it will be done.
Plausible concept; will be interested to see the results from the UAE prototype. Please report back mid-2008 (which is only 6 weeks away).
Some marketing fluff points are a bit hard to swallow, such as wind costing over $.30/kWh and PV never possibly being less than $200/m2. These reduce the level of confidence people will have in your pitch.
I hope that Dr. Hinderling will publish a second guest post with TOD specifically on the prototype. I already invited him to do so.
Maybe you are a bit harsh here in your judgment.
Remember that Dr. Hinderling calculated figure 14 for latitudes below 20o. Most profitable wind turbines are at considerably higher latitudes, where there is more wind. Furthermore, Dr. Hinderling talks about the cost of producing wind power, and not the price at which it is being sold. Many European countries today subsidize alternate energy generation in order to push the technology.
Also, the PV numbers are quite realistic. Remember that this is not the price of the photovoltaic panels alone. You either need to store the energy in golf cart (deep cycle) batteries, or you need to sell to the grid, in which case you need an inverter and a transfer switch.
I built a PV system a few years ago in the White Mountains (=boonies) of New Mexico: 24 Kyocera 80W PV panels (12 mounted on the roof, the other 12 mounted on a ZoomWorks tracker), a Trace inverter, 20 golf cart batteries, and a backup Kohler propane generator. The system produced about 6 kWh of energy per day, and it cost close to $30k, i.e., slightly more than $1k per m2.
Higher volumes and improved technology may bring the cost down by a factor of 4-5, but much more cannot be expected within a reasonable (near-term) time frame.
That's the quote from the article. Nothing about inverters, batteries, etc.
I have a 2kW system now with AGM batteries (Solarex, Outback, Concorde), so I understand the component costs. And I got 3.5 kWh on a mostly rainy, overcast day today.
I like the inland version better because it would not be exposed to some of the problems outlined with waves (a great source of power in their own right)
Here's a possible additional idea -it strikes me that the reflecting 'wedge' could themselves be formed by having an additional thin layer on top of the support layer that had channels in it that could be pumped up with air. Normally this would form cyclindrical peaks but you could put a stiffener in that would make part of the curve flat -the amount of slack that you put into the non-stiffened region would determine the angle of the wedge:
Where '/' = stiffened and reflective coated film and '*' = extra slack film.
............../*
............./+*
............/++*
.........../+++*
____/+++*__________
_____________________Etc-->
'+'=overpressure
The parallel '_' regions are bonded together...
You could mass produce this sort of structure very cheaply then simply unroll it and 'blow it up' to produce hundreds of reflecting ridges of differing angles...
If you didn't want to go down the air pump route you could make the wedges with expanded polystrene...
Nick.
I notice no mention of Ocean Thermal Energy Conversion (OTEC) in these discussions. There is an excellent, if somewhat dated (pub. 1994) book on the subject: "Renewable Energy from the Ocean - A Guide to OTEC", by Avery and Wu, published by the Oxford University Press, as a part of The Johns Hopkins University Applied Physics Laboratory Series in Science and Engineering. Two additional sources of information about OTEC are: "Ocean Thermal Energy Conversion", by Takahashi and Trenka, published by John Wiley and Sons as a part of the UNESCO Energy Engineering Series, in 1996; and, "Renewable Energy - Sources for Fuels and Electricity", edited by Johansson, Kelly, Reddy, and Williams, executive editor Burnham, published in 1993 and contains a wealth of information, somewhat dated, about a wide variety of renewable energy systems.
The concept of OTEC was originated by Arsene D'Arsonval in 1881, and proven by his pupil Georges Claude, in Cuba, in 1930. Additional work has been done since the 1930's, particularly in Hawai'i after the 1970's oil price spikes, but OTEC has yet to be developed on a commercial scale. It is my understanding that one of the bids to repower Diego Garcia is OTEC based, but I have no knowledge of the current status of the bidding process or plant development. Several sources of information about OTEC are available online (do a search with your favorite search engine), one of my favorites is: http://www.seasolarpower.com.
From "Renewable Energy - Sources for Fuels and Electricity":
"The oceans act as an enormous heat sink for solar radiation falling on the earth. About one-fourth of the 1.7x10^17 watts of solar energy reaching the earth's atmosphere is absorbed by sea water ... Overall, OTEC's theoretical potential, if not restricted by economics or technical problems, is about two orders of magnitude greater than that of wind, wave, or tidal energy."
From "Renewable Energy from the Ocean - A Guide to OTEC":
"The area in which the deltaT between the ocean surface and 1000m depth exceeds 22C ... is approximately 60 million square kilometers. An estimate of the bus-bar power that could be generated annually by deployment of OTEC plants in this region at a spacing that would be environmentally acceptable exceeds 10 million MWe (net) ... This would provide on-board OTEC power for potential production of fuel energy of 200 quads/year of ammonia or 640 quads per year of methanol ... These high-octane liquid fuels have been demonstrated to be good alternatives to gasoline. Gasoline consumption in the United States in 1987 was 14 quads of fuel energy."
In tropical locations, with steep bathymetry profiles, OTEC plants can be land based, and there can be additional benefits of district cooling (using the still relatively cold water from the discharge of the OTEC power plant as chilled water...eliminating the most expensive part of an air conditioning plant - the compressor), and fresh water production. Also, at the site of any OTEC power plant, the nutrient rich cold water brought up from the depths can be used for mariculture to produce high quality seafood.
An alternative to production of ammonia or methanol is to produce hydrogen. The hydrogen could then be liquified and transported in LH (Liquified Hydrogen) tankers, similar to the LNG tankers used to transport natural gas in a liquid state.
There are a couple of significant "ifs" here. Economics governs everything, we all want loads of money for not doing very much. The energy density of FF has alowed us to sit on our arses for much of our time. If we all start to do real work again instead of playing a giant game of monopoly we may be in with a chance.
The other possibility is that 'fat boy energy user' has a heart attack...
For more OTEC news visit www.otecnews.org
Nick.
speak for yourself.
FF will just be more highly valued and will be used more efficiently in the future. most people could buy a Prius and almost halve the amount of gasoline they use in a year. that's JUST by buying a Prius and says nothing about car pooling in a Prius, driving your Prius less and walking/biking.
and note in this instance energy use declined drastically yet how much was your life truly impacted for the worst? you exercise a bit more? that's good for you and will EXTEND your quality of life. you car pool and make a few more friends? that's good for you and extends your quality of life. you drive Prius instead of a huge SUV you didn't need? is that bad? you wallet will certainly thank you which will allow you to purchase more food which has gone up in price because of peak oil...
now repeat the scenario in 7 years using a PHEV or an electric vehicle...no reason to be a doomer!
Unfortunately I'm not, It was a metaphor to describe the non productive section of society, which has built up to administer bureaucracy and finance. I actually build things and repair them, keep things going use my bike and drive a small diesel, I also liftshare when possible. Most people could buy a Prius, but most people don't. Infact I have only ever seen one in my life and this was parked up! There can only be one reason for this, they are not as effective as we are lead to believe.
Its not what people could do its what people are doing- nowt.
finance is essentially to society. it may be too much of GDP right now, but it is vital. where would the internet be without it? you may build things and repair them and that's great, but how many people financed the stuff you repair?
as for the Prius, I see those all the time. you can't drive around here and not see one parked or on the road. they are effective considering they have sold about a million of them when just a few years ago most doomers probably thought they were vaoporware...
my relative has a prius by the way.
You mean essential!
I wouldn't disagree with that. The real success of (two give two examples) Edison and Brunell was their ability to gain access to finance, but they also has access to plentiful cheap labour to build the projects they financed.
As many sensible people have pointed out here, its cost that counts. We could probably do anything we want if we could get people to work hard enough for low enough salaries. That as far as I can see is the problem, people want too much output for not enough input. Put more diplomatically than above, oil has allowed this situation to develop.
I don't see how this system can cope with a rainy week without expensive backup. Despite the cost I think distributed PV + microstorage has a lot to commend it. That storage could be a car or house battery such as a beefed up uninterruptible power supply. The huge advantages are no moving parts and multiple connections.
The article suggests PV will always cost at least $200 per square metre. I'll take that as $1 per watt. A poster here suggested electrical storage could get down to $12 per kilowatt hour. However short-life shallow-draw lead acid batteries seem to work out more like $200 per kwh. Anyways if we want A amount of nameplate generation capacity (in kw) and B of storage (in kwh) then the capital cost will be A + 12B.
The problem is that A and B will vary according to sunny or cloudy location and whether we want long term storage such as a week. Big bucks but the payoff is great resilience.
Interesting, but it does not address the liquid fuels problem (food production and transportation), and it will use up much oil, natural gas, and coal. And as soon as oil production begins to decline we go into worsening depression and we will have spare electric power for some time. This solar stuff depends on oil for mining ores, manufacture, transport, and maintenance. Time has run out and there is a lack of capital. Got any other ideas :(
Oh no, no, no cjwirth. There is PLENTY of time.
Didn't you see the good doctor said, "An analysis of future global petrochemical consumption needs ... implies that early petrol shortages might already appear in the mid of the century.
So you see, we've got decades left before we see "early petrol shortages." Sit back and relax. We have plenty of time and resources to fritter and waste an off-hand way.
A bunch of intellectual masterbation.
first of all, peak oil does not mean peak energy and it doesn't mean that we won't mine at all. mining might be scaled back. might be. however, can we not run the mines on solar and wind and whatever other alt energy source we find? is south africa not still mining?
Peak Oil is now, and Peak Natural Gas and Peak Coal are soon to follow, according to ASPO Ireland and the German Energy Watch Group. South Africa, like every nation, is kept afloat with oil. No oil means no energy. Mining will be scaled back a bit, then collapse. Solar stuff IS going up in price, like oil, and soon no one will be able to afford it, neither individuals, business or government. We are where we are. Time to think about conservation, not wasting oil on solar toys that will soon litter the landscape, dead, like the million of cars, SUVs and pick ups.... all monuments to wanting "more, more, more...."
Like oil, the only reason the price is so high is because it is in demand. Demand at historic prices greatly exceeds production capacity, so prices went up.
The result is that solar stuff became very profitable, and is attracting a lot of investment. The cost continues to go down, so the result will be much larger manufacturing volume.
You're confusing price with cost. If no one can afford the product, how are the makers going to sell it? Prices will remain high until production gets closer to demand, at which time they'll moderate; the affordability will depend on the immediate supply/demand situation. About the only thing which would prevent this is a rapid collapse of the industrial economy, where there is no ability to invest.
This is an extremely contorted, confusing and naive POV view E-P.
"cost continues to go down, so the result will be much larger manufacturing volume."
Really? And what if costs do not "continue to go down?" And what happens if, due to chronic "demand destruction" (ie. economic death), there is not sufficient demand to support the ramped-up industry? Will the industry continue to "attract investment" then?
This whole discussion suffers from too many simplistic assumptions and too many "all or none" absolutes (like "rapid collapse" - whatever that might mean to you).
There will continue to be mining - including "solar mining" - at one scale or another indefintely.
But because of petro-gangrene and economic death, fewer and fewer people in more at more places over time will be able to afford the mined products.
PV-grade silicon is coming to market at costs as low as $8/kg. Current costs are driven largely by the overhead of small-volume manufacturing. As volume increases, more automation can be brought to bear. There is an enormous amount of demand which can be shifted. PV production is around 1 GW/year, US peak demand is around 800 GW. Substituting electricity for petroleum might add as much as 500 GW over the 8-hour generating day. What would stop this trend any time soon?
Prices would fall to approximately the cost of production. But the price of energy from these units would be much cheaper than oil, so demand would hold steady.
Don't underestimate the potency of cheap silicon. Silicon at even the $14/kg of the SRI process (Na2SiF6 to Si) comes to about $4/m² of 100-micron silicon, perhaps ten times that in finished panels at high volume. That's under 25¢/peak watt, which in turn yields energy at less than coal. This resembles the situation at the beginnings of the world oil boom, and while there are limits to how much surface we can cover with PV we won't run out of photons to feed them for longer than the history of multicellular life on Earth.
John, you ask good questions for a 15 year-old.
You asked about energy and South African mining - "is not south africa not still mining?"
Well, yes Virginia, they are. But the Real World is a little bit more complicated than that. It's NOT a simple "yes" or "no" question. If you think otherwise, go buy some stock in S. African Mining companies.
I am not a gold mining stock expert but profits could actually increase for the miners if the price of gold rises high because the mines had energy issues...
It seems that one of the big problems the SA miners are having is(was?) the rise of the rand.
I just submitted this to slashdot. Here is the entry, go upmod (click the plus sign after logging in) it if you are so inclined:
http://slashdot.org/firehose.pl?op=view&id=680846
The discussion of materials and capital always fascinate me. Just the other day I was seeing adverts for the new Mercedes C-Class AMG with the V-8 engine. Here we are, supposedly on the eve of the end of enough materials and money to build solar and other renewable energy systems, and Mercedes seems to have procured the material to build 451 horsepower automobiles, and by the way, find customers for them!!
This is only one of a thousand examples I could use. How is there is no material and money for alternative energy projects (NOW, the emergency in getting materials is NOW, we are told again and again), and yet the constructors of planes, luxury power boats, motorcycles, office furniture, electronics and a thousand other material hungry comsumer products goes right on along. One wonders how many million square feet of high quality, high tech optics have been sold in the form of computer monitors and flat screen TV sets in the last few years...and yet, solar will consume electronics, glass or aluminum, it can't be done! How much steel has been poured into the new giant skylines of Dubai and the cities of China, how many miles of new roadway, ports and bridges built? Where is all of this material coming from? It seems we have an iron clad rule: If it is going to consume energy, we have all the materials we need to build it. If it is going to produce energy, sorry, we're out!
RC
Right, RC. I just spent 10 days in the sunny west, on a family reunion/goodby meeting. Very wealthy place, home of VC's. All the people I met were rolling in dough, as they say, and that mercedes was there too. The sky was a perfect clear blue. Nobody had heard of any oil shortage, $4/gal was nothing to them. Energy efficiency? They had a high tech flush toilet. The weather was hot one day, but something called a compressor had crapped out and AC didn't work. The nights were cool but nobody even thought of airing out the house to get it cool at night and then shut it up during day.
A truck pulled up to the tiny yard near by, and a guy got out and used five, count 'em, 5 different roaring gasoline engines to do things like mow and trim, doing the work that I could have done with a few very simple hand tools in a few minutes -running on two spoonsfull of oatmeal.
As for global warming and all that, nothing. And when I asked what all these good people were gonna do when a junk freighter dumped a couple of thousand starving Indians on the beach, one of them, a dear little old lady with a ready wit (and my sister) quipped " kill 'em and feed "em to the servants". Of course she said it for the shock effect, but everybody else was yelling so loud to their equally deaf neighbor that this little sally went unnoticed.
Anyhow, you people are far more enlightened, so it puzzles me that you all don't just say the obvious- the solution to energy is solar thermal in deserts, HVDC to population centers, and pumped hydro both ends of transmission lines. Pay for it by not making those goddam mercedes' for a decade, like in WWII.
Sure, I have said this several times before, but for some reason _(deaf too?) you aren't hearing.
Now, back to the real work. Having a huge amount of fun making-- ha, you guessed it-- Solar thermal machines-- the ones that really work. See ya. Don't talk too much, it"s hard on the vocal cords.
Is this some sort of joke? If only we had the water and materials to make something like this by the hundreds of thousands. Maybe it's a little easier to track the movement of the sun with this, but the water evaporates. And getting enough water and moving it into the desert would take more energy. Desert sand and dust can cloud out the mirrors-- and, of course, it only works in the spring and summer, middle of the day. It only works about 20% of the time. So no matter how cheap, you need storage. A single gallon of gasoline contains the energy content of one ton of lead-acid storage batteries. We need energy storage, which this can't do.
The real answer is fission or fusion. ITER is decades off, but sodium fast reactor technology is mature. Nuclear fission is a virtually limitless energy source using low-grade ores in fast reactors.
Said by Francois Cellier:
The mean insolation of ~250 W/m2/day assumes the PV panel is pointing in a fixed direction. A solar island is a single axis tracking system which tracks in azimuth. To compare equal systems Dr. Hinderling should use a PV panel installed on a single axis tracking system and located within 20 degrees of the equator. The assumption of 10% efficiency should be updated to 15% for modern monocrystalline PV's. Correcting these underestimates would increase the power received and the revenue earned to at least 430 kW*hr/yr and ~$30/m2/yr, approximately double the stated values.
The maximum cost for a PV system compared to crude oil in a competitive market is not a fixed amount. One should consider the rising cost of crude oil and its inability to satisfy demand as it becomes increasingly scarce. Its negative environmental impacts should be included. Does that $.07 /kW·hr equivalent for crude oil also include the cost of the ongoing war in Iraq?
Hey Guys,
I have single handed a yacht around the world and those waves, even way out there get pretty big.
Nobody has lived until they have seen their first forty foot wave.
Those islands will have to be pretty flexible.
At 1.5 km diameter, the height is probably going to be higher than 40 ft. And, there may not be such large waves where these are intended to be used since the weather is not so extreme at the equator.
That must have been a great experience on the yacht.
Chris
Some of the figures seemed strange to me and the clarification that this is pitched to oil rich equatorial nations helps me understand this better. The first number that seemed strange was the assumed price for oil based electricity. At $4.50/gallon for diesel and about 130 MJ/gallon that comes to about $0.30/kWh at 40% efficiency, not $0.07/kWh in the article. Basically anywhere in the world today would do well to replace as much oil generation as possible with solar PV since this can be done quickly. But, for countries that are willing to forgo the profits to be had by selling the oil, the $28/barrel price for oil that is assumed is in some sense reasonable. Also, the idea of shortages at mid-centrury is reasonable if you have a lot of oil now.
Others have mentioned the discrepency on the wind resource, but this is explained as owing to being in the dodrums. This (low wind), together with the substantial surface tension in such an arrangement would tend to make the Kelvin-Helmholtz instability that worries EP pretty minimal. There appears to be active stabilization through the periodically placed over pressure pumps. There is no reason not to have an arrangement of quasi-pressure cells within the sturcture to assist with stabilization. Curtains would probably do the trick. Defocus is not really an issue if both the reflector and the vacuum tube are tilting together which would be the case if the wavelength were large compared to the width of a collector strip.
It is actually cloud cover that is most important for making CSP work rather than latitude, but since cost will go as surface area parallel to the earth for this project, being near the equator helps more than for other types of steering arrangements. The issue of rain is going to be pretty small in a good CSP site but, on the other hand, it would be worth the pumps to collect the potable water that you would get from time to time, so your drainage system would likely pay for itself.
As this goes forward and oil tankers become available owing to reduced oil trade, remote equatorial farms might charge tanker based flow batteries and provide dispatchable power from ports around the world.
Chris
A more efficient and cost effective renewable energy system is needed.
A more efficient and cost effective renewable energy system is needed.
To accelerate the implementation of renewable electric generation with added incentives and a FASTER PAYBACK - ROI. (A method of storing energy, would accelerate the use of renewable energy) A greater tax credit, accelerated depreciation, funding scientific research and pay as you save utility billing. (Reduce and or eliminates the tax on implementing energy efficiency, eliminate increase in Real estate Taxes for energy efficiency improvement).
In California, you also have the impediment, that when there are an interruption of power supply by the Utility you the consumer cannot use your renewable energy system to provide power.
In today's technology there is automatic switching equipment that would disconnect the consumer from the grid, which would permit renewable generation for the consumer even during power interruption.
New competition for the world's limited oil and natural gas supplies is increasing global demand like never before. Reserves are dwindling. These and other factors are forcing energy prices to skyrocket here at home. It's affecting not just the fuel for our cars and homes, but it's driving up electricity costs, too. A new world is emerging. The energy decisions our nation makes today will have huge implications into the next century.
A synchronous system with batteries allows the blending of a PV with grid power, but also offers the advantage of “islanding” in case of a power failure. A synchronous system automatically disconnects the utility power from the house and operates like an off-grid home during power failures. This system, however, is more costly and loses some of the efficiency advantages of a battery-less system.
Jay Draiman, Northridge, CA
May 21, 2008
I think that the House just voted to pass continuation of some renewable energy tax incentives. http://ap.google.com/article/ALeqM5icrolgbXr0mlnZQmLr-1svIyNDpwD90Q858O1
There are ways to make this go even faster.
It is usually possible to get a switch that allows you to use a grid tied system during an outage. You don't have to have batteries if you just want to keep the food in the freezer from spoiling but you would need them for more than that.
Chris
In a grid tied system, you pay for the consumed energy, whereas in a stand-alone system, you pay for the installed power. This can make grid tied systems attractive, as you don't have to provide for the worst case (maximum load).
Grid tied PV systems slowly gain in importance here in Europe with the new building codes. The "minergy-P-Eco" standard almost forces you to go that route. During the day, especially during the summer months, you produce surplus electric power that you feed into the grid (sell to the electric company), and during the nights and in winter, you buy electricity from the grid. Thereby, you leave it up to the electricity company to solve the storage problem for you.
Here in Switzerland, with lots of hydroelectric power, daily fluctuations are compensated for by pumping water uphill into the reservoir lakes during hours of low electricity consumption, and letting the water flow downhill again during hours with high electricity consumption.
If more customers should choose to install grid tied PV systems, the electricity companies will have to invest in more and bigger pumps. Our reservoir lakes are big enough to hold the additional water for much higher daily fluctuations on the production side than we currently see.
However, this system won't be good enough to deal with seasonal fluctuations as well. For that we would need to increase the size of the available reservoir lakes quite a bit by raising the height of the dams.
I was really responding to the question of using a grid tied system when the grid is down. It works when the sun shines (every day after a hurricane for example) and so it can do some things. But, it can't run your TV at night.
For seasonal fluctuations in production where you live, I would think that reducing winter time demand would be the most cost effective thing to do.
Chris
Certainly. The added cost of storage is not all that great by the way. At our weekend house in New Mexico, we had 20 deep cycle batteries, costing $80 a piece, i.e., the cost of storage was $1600 out of a $30,000 installation. The batteries allowed us to run the house for 2 days without firing up the backup generator.
The issue is not primarily cost. It is mostly a question of inconvenience. The batteries required quite a bit of maintenance (regularly checking water levels, periodically regenerating the batteries using the backup generator, cleaning the contacts, etc.) Most people here in Switzerland aren't willing to do this at the current time ... but then again, we don't see many brown-outs yet around here. If the grid should ever become unreliable, more people will be happy to go the extra mile for the convenience of having uninterrupted service at their home.
Absolutely! This is what the new building codes are for. The additional winter demand is primarily caused by heating poorly insulated private homes.
If most of the hassle is maintenance then I wonder if more centrally run flow batteries might do the job?
I'd like to join in on the thanks for the presentation of this exciting new technological idea, and apologise if my responses sounded curmudgeonly.
It is not easy to make a serious proposal which involves transporting energy many kilometres, or turning them into a liquid fuel, and my personal reaction is that I prefer that the system is proved and initially used nearer to where it is produced, and we worry about running larger parts of society on it after we have some sort of proven technology, but in no way wish to pour cold water on attempts to make a relatively clean energy source.
Displacement of some of then NG burn in the Gulf would seem to be a good initial target after prototyping.
Are you referring to new building codes in Switzerland? If so, how close are they likely to come to eliminating additional winter demand?
First of all, the new building codes aren't mandated yet here in Switzerland. They are only labels, similar to energy ratings of your refrigerator. They specify, how much heating power your house consumes on average per m2 of heated area.
A "minergy"-rated house consumes less than 40% of the heating power of of the average traditional house. Minergy rating is being achieved by two measures. On the one hand, a minergy house uses better insulation for walls, roofs, and windows, and on the other hand, it uses controlled air flow with a heat exchanger for ventilation. Many (most?) new dwellings here in Switzerland are now built to minergy standard.
A "minergy-P"-rated house reduces the energy consumption further by another 25% below "minergy"-rating (i.e., it consumes less than 30% of a traditional house). There are no special measures taken to achieve minergy-P rating. It was simply recognized in recent years that the minergy standard had been too relaxed, i.e., more can be achieved than had originally been considered possible. Minergy-P-rated houses are still rare here in Switzerland. Yet, whereas the standards had been developed here in Switzerland, our neighbors to the East, in Vorarlberg (Austria), are quite a bit ahead of us in building to these new standards.
A "minergy-P-eco"-rated house reduces the external energy consumption down to zero. This can only be accomplished by producing energy locally, usually by placing PV panels on the roof of your house. These are grid-based systems, i.e., they sell on average as much electricity to the grid as they buy from it. Other minergy-P-eco designs are possible as well. A colleague of mine here at ETH Zurich is currently constructing a new house by placing a serpentine of warm-water pipes into the outside walls. Thereby the house "thinks" that it is being located in Sicily, where no heating system is needed. The warm water is being produced by a geothermal system.
By building to these new standards, it should be feasible to reduce the additional winter load over the years by at least 30%. More is difficult due to the many older buildings that we have here in Switzerland that are under monument protection.
I don't think that retrofitting is going to turn out to be as hard as we've been thinking. This type of window: http://www.nextenergynews.com/news1/next-energy-news2.27b.html may be energy positive even on the north facing part of a building. That is probably half the battle. Making the stuff look like the glass it replaces in your monuments would actually be a fun project to undertake.
Chris
I would like to comment on some of the remarks that were made.
1. It seems that I did not explain very well the concept of the floating, or "hovering" of Solar Islands. The ring will carry a platform-membrane which will float by a slight overpressure below it. If there is no wind and the surface loading is equal everywhere on the platform, then there are no horizontal forces, and there is no horizontal tension on the membrane. The membrane hovers high above the waves (~20 m), so that they do not touch the membrane (at least at wind-speeds up to 70 km/h, according to our simulations). Of course, Solar Islands will have a satellite-guided weather alarm system, so that they can avoid the worst storms.
2. The Solar Island concept allows for thermo solar concentrators (CSP), but also for photovoltaic concentrators. In the second case (concentrations of up to seven suns), it acts like a very low-cost 2d-tracking system, and is thus providing extremely low-cost photovoltaic energy.
3. I agree that PV is better suited for northern regions, CSP more for southern (resp. near the equator) regions. But I see a different reason for this: Very large solar plants do not make any sense north of say 40° (just for reasons of profitability). But it is well possible that many small plants will be built. PV is clearly superior for smaller plants, just as CSP is superior for very large plants. Therefore, on the northern hemisphere, PV will be more in the north, CSP in the south (vice versa in the southern hemisphere).
4. I fully agree to the necessity of energy storage. There are two types to consider: Seasonal storage and daily storage. Daily storage is relatively easy and can be done in several ways: Thermal storage, chemical storage, electrical storage, and others. The best one will be used! This problem is common to all solar energy sources (i.e. no sun at night). Trickier is the seasonal storage. In the northern countries, most energy is being used in wintertime, and it is just then that solar energy is hard to get (the low angle of the sun, the short duration of the day and the high probability of overcast sky). If solar energy is to play an important role in the world-wide energy supply, then there is no other solution than to bring the energy from the south to the north (at least during wintertime), in the form of long distance high voltage lines, but more probable, in the form of solar fuel (i.e. hydrogen or other chemical carriers). It seems to me that only chemical carriers would have the necessary energy densities, but I might be wrong.
Many thanks to all the interesting contributions!
Thomas Hinderling
Thanks for joining the discussion and thanks also for the article. Taking your comments in order:
1) From what you say about deflection of less than 20 meters for a wind speed less than 70 km/h, over 2.5 km this would be a tilt of about half a degree or about the diameter of the Sun in the sky. Thus, even if you get this much tilt, and your absorber were exactly matched to the Sun's size without tilt, you would only lose half of the power from very high wind. I could be misreading you here and you are saying that the waves don't get to 20 meters rather than the membrane does not dip to 20 meters.
2) With ocean water available for cooling, I would think that you could go to higher concentration for PV. In at least some cases, this looks as though it would be worth the effort: http://mdsolar.blogspot.com/2008/03/lux-lucis-tepida.html
3) It seems to me that the profitability of large solar plants is not all that dependent on latitude except in that there are fewer deserts at higher latitude. That it, it is not the effect of airmass but clouds that reduces the annual energy available to concentrating systems to a larger degree. You need more land at higher latitude, but not more equipment in the absence of the cloudiness. There are spots in Montana where CSP might make sense: http://www.nrel.gov/gis/images/us_csp_annual_may2004.jpg Airmass does have some effect though.
4) One aspect of solar fuel produced seasonally is that we already have the infrastructure to deliver it in the form of oil and gas tankers. They do the job now in fact. The edge support ring in your design seems to me to be a place where industrial processing might take place to produce and store fuels. I know the US navy is hopeful about using carbon dioxide that is disolved in seawater as a source for hydrocarbon fuel production. If you are running desalinization with you waste heat, you might be processing enough seawater to get some carbon feedstock as a coproduct and direct this to fuel production. Of course, if tankers are visiting an island, they could always deliver carbon dioxide captured from land based industrial processes such as calcination for cement making.
Again, thanks for the post.
Chris
Thanks for your comments. If I may reply:
to 1):
What I wanted to say is that the waves are (usually at least) less than 20 m high and therefore would not touch the membrane. The platform will be moving at the rim - but even there, the mean distance from the sea level remains absolutely constant (like a vibration on a string - the mean amplitude is zero). So you will observe movements at the outer rim which are diminishing towards the inner regions of the island. These movements reduce the efficiency of the focussing. However, our simulations have shown that even in relatively high wind situations the loss of efficiency is small. However, simulation is not reality, I know!
to 2):
I completely agree - the optimum is to be found on the basis of a cost / performance analysis.
to 3):
There is another weakness of CSP in the northern latitudes: CSP can not really be envisaged in a 2d tracking mode. The difference between 2d tracking (azimuth and elevation) and 1d (only elevation or only azimuth)tracking is the higher the more you go north. Secondly (and this is probably what you are saying, too), CSP is only converting direct radiation, not diffused light. But diffusion is more important at low elevations than at high elevations, therefore more important in northern latitudes.
to 4): I could not agree more! Using the Sabatier effect, one could produce for instance methane from H2 and CO2 using heat. Methane is much easier to transport than hydrogen. Burning up the methane generates CO2 - but not one molecule more than was used before to convert H2 into Methane!
Best regards
Thomas
1) What I understand now is that vibrations from waves transfered from the support ring are more important than Kelvin-Helmholtz instability induced ripples from wind shear over the surface of the menbrane. This makes perfect sense since the fetch for the waves is much much longer than the diameter of the membrane. Again, just dimensionally, the tilting of the reflector-absorber set up can do all that much to defocus since the thing would have to be sinking to get much of an angle.
3) 2-D tracking is pretty much part of solar tower or sterling dish approaches. In terms of diffusion, there is Rayleigh scattering, areosols and clouds. Clouds really have the largest effect. But, for two regions with the same cloudiness but different average solar elevations, the one with the lower elevation will have a greater effect from clouds because the typical line-of-sight to the Sun will incercept more of them. The other two effects are combined in the term airmass which astronomers use to correct for atmospheric extinction.
4) The Sabatier reaction (and Fischer-Tropsch for that matter) is exothermic. So, what you really need is a good use for the heat from the reaction. Otherwise, your efficiency is pretty low since you throw away much of the energy you put into cracking water. Again, desalinization might come to the rescue in this situation. You also get some ultra-pure water from the reaction itself which might have some added value.
Chris
Define 'much'. The Sabatier reaction is:
CO2+4H2 ==> CH4 + 2H2O
The HHV of 1 mol of CH4 is 79% of the HHV 4 mol of H2. Methanol synthesis via:
CO2+3H2 ==> CH3OH +H2O
is even more attractive since the HHV of 1 mol of CH3OH is 87% of the HHV of 3 mol of H2.
However, a problem with either of these processes is obtaining low cost supplies of CO2. No one has convinced me that CO2 can be removed from the atmosphere at reasonable costs (in energy and other terms). Sequestering CO2 at the site where the fuel is burned and then transporting it to the site where hydrogen is generated is also likely to be quite expensive. Ammonia (NH3) is another possible energy carrier avoids the problems mentioned above (although it may have other problems) because of the abundance of nitrogen in the atmosphere relative to CO2. Nitrogen is produced via:
3H2+N2 ==> 2NH3
The HHV of 2 mol of NH3 is 91% of the HHV of 3 mol of H2.
OK, if you want to just throw away 22% of the energy go ahead. At my house we eat everything on our plates ;-o
Pretty clearly, one can get pure CO2 (pure enough to avoid nitrogen poisoning) from cement manufacture. That is probably enough to handle aviation fuel use. When making hydrogen, there is a pure stream of oxygen which could be used for biofuel combustion allowing for collection of the pure exhaust stream. But, it seems to me that adopting the pressure swing method used on the space station to capture CO2 from the atmosphere is not all that energetically costly. That relies on zeolite: http://mdsolar.blogspot.com/2007/12/jet-fuel.html
If you look starting at about p. 64 of the thesis linked here: http://stinet.dtic.mil/oai/oai?&verb=getRecord&metadataPrefix=html&ident...
You'll see that getting CO2 from sea water takes little energy and the pumping energy might be integrated with the control (turning) of the solar island in this application. In all, I think that getting CO2 for a feedstock is not that large of a challenge.
Good comment!
Chris