Space-Based Solar Power
Posted by Rembrandt on March 21, 2012 - 11:39am
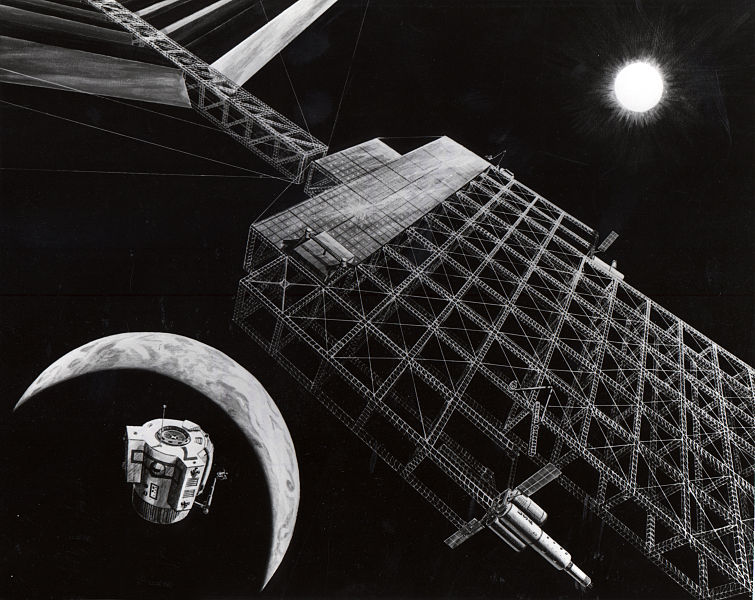
I’ll take a break from writing about behavioral adaptations and get back to Do the Math roots with an evaluation of solar power from space and the giant hurdles such a scheme would face. On balance, I don’t expect to see this technology escape the realm of fantasy and find a place in our world. The expense and difficulty are incommensurate with the gains.
How Much Better is Space?
First, let’s understand the ground-based alternative well enough to know what space buys us. But in comparing ground-based solar to space-based solar, I will depart from what I think may be the most practical/economic path for ground-based solar. I do this because space-based solar adds so much expense and complexity that we gain a large margin for upping the expense and complexity on the ground as well.
For example, transmission of power from space-based solar installations would likely be by microwave link to the ground. If we’re talking about sending power 36,000 km from geosynchronous orbit, I presume we would not balk about transporting it a few thousand kilometers across the surface of the Earth. This allows us to put solar collectors in hotspots, like the Desert Southwest of the U.S. or Northern Africa to supply Europe. A flat panel tilted south at latitude in the Mojave Desert of California would gather an annual average of 6.6 full-sun-equivalent hours per day across the year, varying from 5.2 to 7.4 across the months of the year, according to the NREL redbook study.
Next, surely we would allow our fancy ground-based panels to articulate and track the sun through the sky. One-axis tracking about a north-south axis tilted to the site latitude improves our Mojave site to an annual average of 9.1 hours per day, ranging from 6.3 to 11.2 throughout the year. A step up in complexity, two-axis tracking moves the yearly average to 9.4 hours per day, ranging from 6.8 to 12.0 hours. We only gain a few percent in going from one to two axes, because the one-axis tracker is always pointing within 23.5° of the direction to the sun, and the cosine projection of this angle is never less than 92%. In other words, it is useful to know that a simple one-axis tracker does almost as well as a more sophisticated two-axis tracker. Nonetheless, we will use the full-up two-axis performance against which to benchmark the space gain.
On a yearly basis, then, getting continuous 24-hour solar illumination beats the California desert by a factor of 2.6 averaged over the year, ranging from 2.0 in the summer to 3.5 in the winter. One of my points will be that launching into space is a heck of a lot of work and expense to gain a factor of three in exposure. It seems a good bet that it’s cheaper to build three times as many panels and stick them on the ground. It’s not rocket science.
For technical accuracy, we would also want to correct for the atmosphere, which takes a 21% hit for the energy available to a silicon photovoltaic (PV) on the ground vs. space, using the 1.5 airmass standard. Even though the 1347 W/m² solar constant in space is 35% larger than that on the ground, much of the atmospheric absorption is at infrared wavelengths, where silicon PV is ineffective. But taking the 21% hit into account, we’ll just put the space gain at a factor of three and call it close enough.
What follows can apply to straight-up PV panels as collectors, or to concentrated reflectors so that less photovoltaic material is used. Once we are comparing to two-axis tracking on the ground, concentration is on the table.
Orbital Options
Are we indeed dealing with 24 hours of exposure in space? A common run-of-the mill low-earth-orbit (LEO) satellite orbits at a height of about 500 km. At this height, the earth-hugging satellite spends almost half its time blocked from the Sun by the Earth. The actual number for that altitude is 38% of the time, or 15 hours per day of sun exposure. It is possible to arrange a nearly polar “sun synchronous” orbit that rides the sunrise/sunset line on Earth so that the satellite is always bathed in sunlight, with no eclipsing by Earth.
But any LEO satellite will sweep past the ground at over 7 km/s, appearing for only 2 minutes above a 30° elevation even for a direct overhead pass (and only about 6 minutes from horizon to horizon). What’s worse, this particular satellite in a sun-synchronous orbit will not frequently generate overhead passes at the same point on the Earth, which rotates underneath the orbit.
In short, solar installations in LEO could at best provide intermittent power to any given site—which is the main rationale for leaving the ground in the first place. Possibly an armada of smaller installations could zip by, each squirting out energy as it passes by. But besides being a colossal headache to coordinate, the sun-synchronous full-sun satellites would necessarily only pass over sites experiencing sunrise or sunset. You would get all your energy in two doses per day, which is not a very smooth packaging, and seems to defeat a primary advantage of space-based solar power in avoiding the need for storage.
Any serious talk of solar power in space is based on geosynchronous orbits. The period of a satellite around the Earth can be computed from Kepler’s Law relating the square of the period, T, to the cube of the semi-major axis, a: T² = 4π²a³/GM, where GM ≈ 3.98×1014 m³/s² is Newton’s gravitational constant times the mass of the Earth. For a 500 km-high orbit (a ≈ 6878 km), we get a 94 minute period. The period becomes 86400 seconds (24 hours) at a ≈ 42.2 thousand kilometers, or about 6.6 Earth radii. For a standard-sized Earth globe, this is about a meter from the center of the globe, if you want to visualize the geometry.
A geosynchronous satellite indeed orbits the Earth, but the Earth rotates underneath it at like rate, so that a given location on Earth always has a sight-line to the satellite, which seems to hover in the sky near the celestial equator. It is for this reason that satellite receivers are often seen tilted to the south (in the northern hemisphere) to point at the perched platform.
Being so far from the Earth, the satellite rarely enters eclipse. When it does, the duration will be something like 70 minutes. But this only happens once per day during periods when the Sun is near the equatorial plane, within about ±22 days of the equinox, twice per year. In sum, we can expect shading about 0.7% of the time. Not too bad.
Power Transmission
Now here’s the tricky part. Getting the power back to the ground is non-trivial. We are accustomed to using copper wire for power transmission. For the space-Earth interconnect, we must resort to electromagnetic means. Most discussions of electromagnetic power transmission centers on lasers or microwaves. I’ll immediately dismiss lasers as impractical for this purpose, because clouds block transmission, because converting the power into electricity is not as direct/efficient as it can be for microwaves, and because generation of laser power tends to be inefficient (my laser pointer is about 2%, for instance, though one can do far better).
So let’s go microwave! For reasons that will become clear later, we want the highest frequency (shortest wavelength) we can get without losing too much in the atmosphere. Below is a plot generated from an interactive tool associated with the Caltech Submillimeter Observatory (where I had my first Mauna Kea observing experience). This plot corresponds to a dry sky with only 2.0 mm of precipitable water vapor. Even so, water takes its toll, absorbing/scattering the high-frequency radiation so that the fraction transmitted through the atmosphere is tiny. Only at frequencies of 100 GHz and below does the atmosphere become nearly transparent.
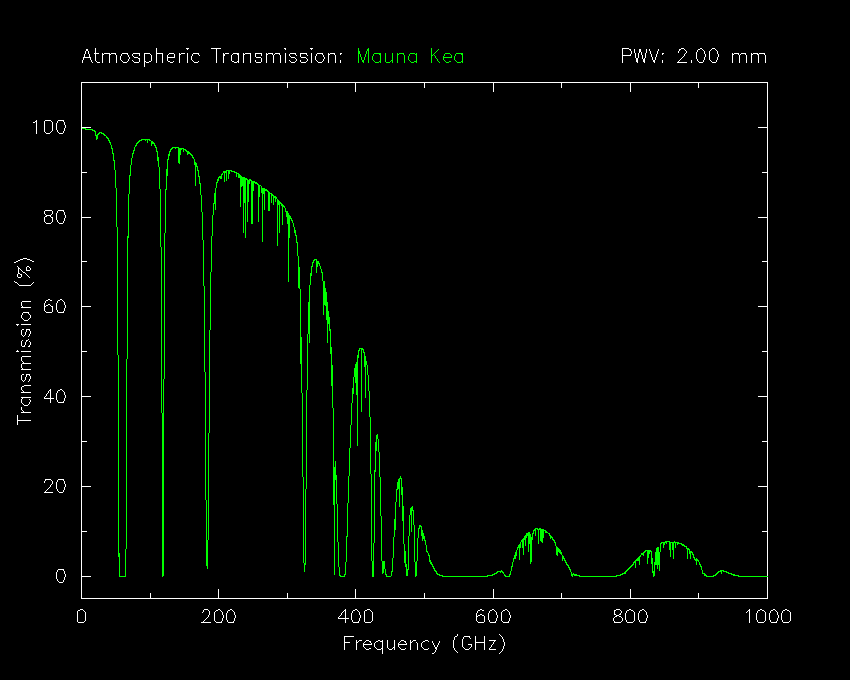
But if we have 25 mm of precipitable water (and thick clouds have far more than this), we get the following picture, which is already down to 75% transmission at 100 GHz. Our system is not entirely immune to clouds and weather.
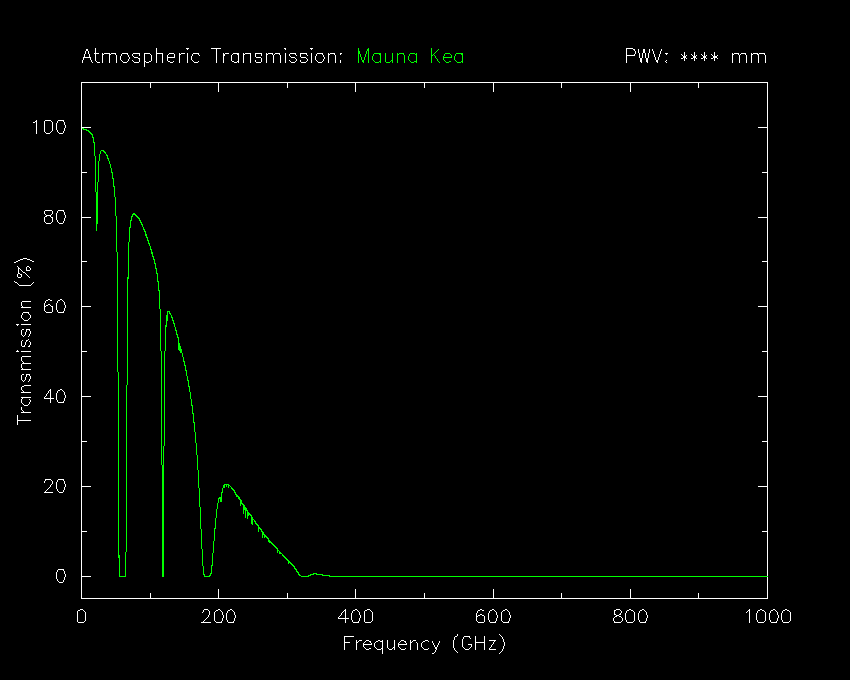
But we will go with 100 GHz and see what this gets us. Note that even though microwave ovens use a much lower frequency of 2.45 GHz (λ = 122 mm), the same dielectric heating mechanism operates at 100 GHz (peaking around 10 GHz). In order to evade both water absorption and dielectric heating, we would have to drop the frequency to the radio regime.
At 100 GHz, the wavelength is about λ ≈ 3 mm. In order to transmit a microwave beam to the ground, one must contend with the diffractive nature of electromagnetic radiation. If we formed a perfectly collimated (parallel) beam of microwave energy from a dish in space with diameter Ds—where the ‘s’ subscript represents the space segment—we might naively anticipate the perfectly-formed beam to arrive at Earth still fitting in a tidy diameter Ds. But no. Diffraction imposes an angular spread of about λ/Ds radians, so that the beam spreads to a diameter at the ground, Dg ≈ rλ/Ds, where r is the distance between transmitter and receiver (about 36,000 km in our case). We can rearrange this to say that the product of the diameters of the transmitter and receiver dishes must approximately equal the product of the propagation distance and the wavelength: DsDg ≈ rλ
So? Well, let’s first say that Ds and Dg are the same. In this case, we would require the diameter of each dish to be 330 m. These are gigantic, especially in space. Note also that really we need Dg = Ds + rλ/Ds to account for the original extent of the beam before diffraction spreads it further. So really, the one on Earth would be 660 m across.
Launching a microwave dish this large should strike anyone as prohibitively difficult, so let’s scale back to a more imaginable Ds = 30 m (still quite impressive), in which case our ground-based receiver must be 3.6 km in diameter!
Now you can see why I wanted to keep the frequency high, rather than dipping into the radio, where dishes would need only get bigger in proportion to the wavelength.
Converting Back to Electrical Power
At microwave frequencies, it is straightforward to directly rectify the oscillating electric field into direct current at something like 85% efficiency. The generation of beamed microwave energy in space, the capture of the energy at the ground, then conversion to electrical current all take their toll, so that the end-to-end process may be expected to have something in the neighborhood of 50% efficiency.
Beam Safety and Consequences
I don’t worry too much about keeping the beam from veering off the collection region. There are clever, fail-safe schemes for ensuring proper alignment/pointing. According to the Wikipedia page on the topic, the recommended transmission strength would be 230 W/m² in the center of the beam. This is about a quarter the strength of full sunlight, and is thought to be a safe level through which aircraft and birds can fly.
At this level, our 3.6 km diameter collecting area would generate about 40 GWh of energy in a day, at an assumed reception/conversion efficiency of 70%. By comparison, a flat array of 15%-efficient PV panels occupying the same area in the Mojave Desert would generate about a fourth as much energy averaged over the year. So these beaming hotspots are not terribly more concentrated than what the sunlight provides already. Again, I find myself scratching my head as to why we should go to so much trouble.
Launch Costs
This brings us to the tremendous cost of launching stuff into space. Today’s cost for putting stuff into geosynchronous orbit is about $20,000 per kilogram of launched material. We have a history of hope and optimism that launch costs will plummet in the future. So far, that has not really happened, and rising energy prices are not going to help drive costs ever lower. Meanwhile, the U.S. space program appears to be scaling back.
In 1999, NASA initiated a $22 million study investigating the feasibility of space-based solar power. Among their conclusions was that launch costs would need to come down to $100–200 per kg to make space-based solar power economically competitive. It is hard to imagine accomplishing a factor-of-100 reduction in launch costs.
Let’s do our own quick analysis. A standard rooftop panel delivers about 10 W per kilogram of mass (slightly better than this, but I will stick to round numbers). Let’s say a light-weighted version for space achieves an impressive factor-of-100 improvement: same power for 1% the mass. This gives 1 kW/kg. I might be grossly over-optimistic in this estimate, but we’ll see where it takes us. Ignoring other infrastructure overhead (wiring, propulsion systems, structural support, microwave transmission antenna, communications, etc.), we end up with a launch cost of $40 per delivered Watt, accounting for 50% delivery efficiency—and this is just the launch cost. I’ll bet the space-qualified ultralight PV panels are not as cheap as the knock-about panels we put on our roofs for $2/W. So maybe the cost of the space hardware, launch of all systems, and build-out of expansive ground systems will cost upwards of $60/W—becoming $400/W if we don’t manage the 100× weight reduction per Watt, settling for 10× instead. Granted, the constant illumination provides a factor of three in favor of space, so we can give it a 3× discount for its full-time contribution. But still, compared to typical ground installation costs at $5/W, we find that the solar approach is at least four times more expensive. You can even throw in batteries in the ground system without exceeding the space cost, and all the reasons for going to space have melted away.
Energy Return on Energy Invested
My initial reaction to the notion of flinging solar panels in space was that the energy needed to launch panels to geosynchronous orbit might totally undermine the energy delivered by such a system. Let’s take a quick look with approximate numbers.
First, today’s silicon solar panels return their investment of energy after 3–4 years of deployment. Stick them in the sun for 30–40 years, and you have an EROEI of 10:1. Specially light-weighted space panels will likely require more energy to make per kilowatt, but will spend a much greater fraction of their time in space soaking up energy. Let’s just guess that the payback would be 5 years if the space panel were deployed on the ground. But in space, the panel works five times longer per day than the panels for which the 3–4 year payback is calculated. So let’s call it an even one year for manufacture payback in space. Panels in space will be subjected to a much harsher cosmic ray (and damaging debris) environment than those on the ground, so we should reduce the lifetime to, say, 20 years. Still, that’s a 20:1 EROEI for the manufacturing piece alone. But then there’s the launch.
A study of gross weight of rockets compared to payload delivered to geosynchronous orbit reveals a roughly 100:1 ratio. This intuitively makes sense to me given the logarithmic rocket equation: much of the fuel is spent lifting the fuel that must be spent to lift more fuel, etc. (see the appendix of the stranded resources post for my explanation of this).
There is a nice rule of thumb—highly approximate—that the embodied energy in products is about the same as that of the equivalent mass of gasoline, at about 40 MJ/kg. Aluminum production requires more, at 220 MJ/kg, but many materials are surprisingly close to this value (and fuel will be right on the mark). A rocket will use a lot of aluminum, but much more fuel. So we might go with a round number like 50 MJ per kg.
If I take my ultra-lightweight panel producing 1 kW/kg, I must launch 100 kg of rocket, at a cost of 5 GJ. A 1 kW panel will deliver 0.5 kW to the end-user, after transmission/conversion losses are considered. The 5 GJ launch price tag is then paid off in 107 seconds, or about one third of a year. Add the embodied energy of the other components in space and on the ground, and I could easily believe we get to a year payback—now bringing the total (manufacture plus launch) to two years and an EROEI around 10:1. If my 100× light-weighting proves to be unrealistic, and we can only realize a factor of ten improvement over our rooftop panels, the solar panel launch cost climbs to three years, so that adding other components results in perhaps a 4:1 EROEI.
In the end, the EROEI is not as prohibitive as I imagined: it’s not a net energy drain as I might have feared. But it’s not obviously better than conventional solar either.
In Summary
I sense that people have a tendency to think space is easy. We have lots of satellites, we’ve gone to the Moon (remember that?!), we used to have a space shuttle program, and we have seen many movies and television shows set in space. But space is a very challenging environment, and it is extremely costly and difficult to deliver things there. If you go to the Fed-Ex site to get delivery costs, you immediately get hung up on not knowing the postal-code for space. Once in space, failures cannot be serviced. The usual mitigation strategy is redundancy, adding weight and cost. A space-based solar power system might sound very cool and futuristic, and it may seem at first blush an obvious answer to intermittency, but this comes at a big cost. Among the possibly unanticipated challenges:
- The gain over the a good location on the ground is only a factor of 3 (2.4× in summer, 4.2× in winter at 35° latitude).
- It’s almost as hard to get energy back to the ground as it is to get the equipment into space in the first place.
- The microwave link faces problems with transmission through the atmosphere, and also flirts with roasting ducks on the wing.
- Diffraction of the downlink beam, together with energy density limits, means that very large areas of the ground still need to be dedicated to energy collection.
Traditional solar photovoltaics in good locations can accomplish much the same for much reduced cost, and with only a few times more land than the microwave link approach would demand. The installations will be serviceable and will last longer. Batteries seem an easier way to cover storage shortcomings than launching stuff to space. I did not even address solar thermal schemes in this post, which competes well with photovoltaics and can very naturally build in storage capability.
I am left puzzled as to why we would want to take a harder, more expensive road to solar power. I think it is just not intuitive to most how difficult and expensive space is. And perhaps they think it’s very futuristic and cool to push our power generation out to space: it fits the preferred narrative about where we’re going. I don’t know—I’m just guessing.
Astronomers frequently face this issue: should we build a telescope/observatory on the ground, or launch something into space? The prevailing wisdom is that if the science can be accomplished on the ground, then by golly you’d best do it that way. You’ll have the result sooner, at less expense, and with a greater chance of success. The lion’s share of astronomical advance is carried out from the ground. Space is reserved for those places where there is no other way. The atmosphere blocks many interesting wavelengths, creates turbulence that makes high-resolution imaging difficult, and produces variations in transmission that make it impossible to measure fluxes to high precision. The rotating Earth gets in the way of continuous observation of a single target for long periods. Some of the more exciting (an well-publicized) discoveries come from space missions, because these avenues are not generally available to us, increasing discovery potential.
Space-based solar power contains little intrinsic advantage that we can get “only from space.” It looks like a wash at best, and the astronomers would say “don’t bother.”
On balance, I don’t expect to see this technology escape the realm of fantasy and find a place in our world. The expense and difficulty are incommensurate with the gains.
Exactly - TOD did this dance once before.
http://www.theoildrum.com/node/5306#comment-494573 (one can still find the original paper I referenced cached)
http://www.theoildrum.com/node/4336?page=1
The "need" for space based power is based on the "need" for 24x7 electrical power. While 24x7 power is an actual need for processes where if you cycle the heat or even rotational status the process breaks/incurs a cost that would not be there if the cycling did not happen most of what is being declared as "need" is really a "want" or even what one had in the past and therefore expects in the future.
Of course 24-hour power is a need. Any replacement for our current power-generation infrastructure has to provide service that's just as good as what we use now, or we'll just burn more coal instead. People want to save the environment, but they want the lights to stay on _more_.
Of course 24-hour power is a need.
Lets say that is true. Lets go so far as to say it is a human right.
Where are the public comments/outcry on how Baghdad used to have a power grid that provided power far closer to 24x7 than after the bombing/invasion?
If 24x7 power was a defensible human right, where is the outcry over the power grid of Iraq? Lybia?
Well, you just said it. We WANT 24/7 power.. it sure would be nice.. some people will be highly inconvenienced without it.. there are surely SOME who couldn't even live without it.. but that really isn't everyone. Having it's just become so unquestioned that everyone in the industrialized world simply THINKS they will die without it.
It's not really about the lights in people's homes, it's more about industrial processes. However, large industrial customers do not get guaranteed 24/7 electricity anyway; on days where less electricity is available (e.g. because of lower water level in rivers), they may be required to turn their manufacturing processes down or even completely off by the regulatory agency. The decision which customer exactly has to reduce their consumption is based on a complex catalogue of criteria, depending on their specific manufacturing processes. Industrial customers are already used to these kind of regulations which have been in place for a long time.
The purpose of these regulations is, of course, to maintain the stability of the grid and to provide 24/7 electricity to private customers. The push for so-called "smart meters", which turn on washing machines and other energy-intensive devices in people's homes at specific times, is to extend this kind of electricity management to private customers. That's why they're being promoted so heavily by electricity companies and regulatory bodies.
Tom has a fine article, but makes a wide variety of fundamental mistakes in his SSP assessment. Here are three to start with:
"Specially light-weighted space panels will likely require more energy to make per kilowatt, "
SSP will likely use thin-film photovoltaics, such as amorphous or CIGS, which are one hundredth as thick as conventional crystalline silicon cells and so thin-film cells require only one hundredth as much energy to manufacture compared to conventional crystalline silicon cells.
Amorphous cells can also be re-annealed (refreshed) while still in space, this was demonstrated on MIR and so they may have very long lifetimes, possibly exceeding thirty years. An introduction to such thin-films can be found here
He also states,
"Let’s say a light-weighted version for space achieves an impressive factor-of-100 improvement: same power for 1% the mass. This gives 1 kW/kg. I might be grossly over-optimistic in this estimate, .."
Actually, amorphous cell thin-films with performance in lab demonstrations at 16.8 Kw/Kg have previously been demonstrated and are now being made multi-layer to improve that performance.
Finally, speaking Aug. 2, 2011 at the AIAA/AMSE/SAE/ASEE Joint Propulsion Conference in San Diego, Elon Musk, CEO of SpaceX, talked about his plan to get launch costs to $50 to $100 per pound by making the Falcon 9 Heavy fully reusable. The Navy's EMALS is another technology now in production that will move electromagnetic launch forward, further lowering launch costs.
Actually he's been kindly optimistic. 100x the power to weight? Rubbish, the big need for kg material in a panel is for structural support and protective transparent cover.
In space, a very strong structural support and transparent cover are needed to deal with space dust impact and such.
I highly doubt you could get even 5x weight improvement over monocrystalline solar panels.
You can't go and clean the space dust off the panels, either, so your panels end up clogged and Swiss-cheesed. Cosmic rays leave their marks as well... solar panels in space don't have as along a life as on earth.
Space solar power beaming down onto earth really is, just as absurd as it sounds.
Good analysis. The one thing missing from your Mohave model though is the loss of power due to long distance transmission. Of course, you will have transmission loss from the space based power also from the point of regeneration to where it's consumed.
The cost to GEO is now more like $4000/kg with SpaceX rather than $20,000/kg with the government. The best place for space based solar may be the surface of the moon. Building materials are local.
Then there's 14 days of sunshine followed by fourteen days of dark.
Yes, it would require three stations around the moon wired together.
Solar panels in space get three times as much sun as solar panels on earth. So we'll establish three stations on the moon so that one of them will always be in full sunshine. The advantage of this is ... I'm not sure.
You realize we can do this on earth. Establish three power stations 120 degrees apart and wire them up. Voila, 24 hours a day solar power. Losses in DC power lines are 3% per 1000 km and half way around the planet is 20000 km.
Of course, if you're already on the moon, you could probably just build your collector(s) up at the Poles, and have them track the Sun around the Horizon in a continuous 360..
But the topic usually departs for me up at that 'if' anyway..
advantages
1) every day is a sunny day
2) no displaced farmland, cities, etc.
3) free land, zero cost for land, free materials (silicon)
4) 6000km for half way around so at 3% per we have 18% loss
Those sound great.
Now what do you see as the Disadvantages?
It's fine to accentuate the positives, but you have to be really clear that the negatives aren't going to eliminate you (Your plan, that is) before you manage to eliminate them.
I listed some in the last article I did on this topic for The Oil Drum
http://www.theoildrum.com/node/7898
They have some disadvantages, however:
* For optical reasons, they don't scale down to small sizes; 5 GW is about as small as you want to make one. [8]
* At 50% loss electricity-in space to electricity-on-the-ground, the cost is doubled from one cent per kWh to two. On the other hand, that's 40 times less cost than transmitting the same power over wires for the same distance.
* They take a large investment to get the cost of transporting parts to GEO down to where they make economic sense.
"..to get the cost of transporting parts to GEO down to where they make economic sense."
Keith,
I have a very hard time believing this key link in the chain is not just thoroughly unhinged by the receeding horizons of our Global Situation(s).. Climate, Energy and Economy .. to name the biggest three heads on the beast, and the same ones that make the other Capital and Government intense energy source so fraught in that future, Nuclear Fission..
To expect to develop a fleet of Massive Lifting Vehicles in the Environment that we are quickly moving into, (an environment that, if the premise of this Site is right, and that has been well anticipated, is not going to head back to the Industrial-Era's Eden from which it has just escaped..)
I don't object to its research, and a set of substantial demos and tests would be welcome to see and review.. but I really don't expect this bumblebee to fly.
"I have a very hard time believing this key link in the chain is not just thoroughly unhinged by the receeding horizons of our Global Situation(s).. Climate, Energy and Economy"
You may be right. My interest in this is showing that there is at least one solution to the problems you cite. To whatever extent climate is being affected by CO2, this is a project that scales to the point it could virtually eliminate fossil fuel use by simply under pricing fossil fuels. The models show that it makes energy at a low enough cost that off peak power could be used to make synthetic gasoline for a dollar a gallon. And this level of energy input could solve the economic problems in much the same way cheap oil for the last century has fueled economic growth.
You do need a fleet of vehicles, 150-200, but they are not very big, less than half the mass of the lightest Boeing 747.
"but I really don't expect this bumblebee to fly."
Can't say I do either, but it's not a matter of money. The US will spend ten times as much on the next war in the mid east as it would take to do this. China, on the other hand, might do it and besides solving their part of "Climate, Energy and Economy" they would get military hegemony because nobody is going to argue with a multi GW propulsion laser.
$4k/kg .. ?
They have yet to prove this in an ongoing working environment.
True, SpaceX has not flown the Heavy yet ($4k/kg GEO), but they have flown the same technology class on the Falcon 9 ($13k/kg), several times and successfully. They also have dozens of launches booked 2012-2017 with NASA, Irridium, AsiaSat, Israel, etc.
http://www.spacex.com/F9-001.php
http://www.spacex.com/launch_manifest.php
http://space.alglobus.net/papers/FetterResponse.html
the Japanese Ikaros satellite, a solar sail, is producing power at about 0.8 kg/kw for the power production system. This extremely low value is accomplished by using a heliogyro thin film solar sail design, which has no structure, and thin film solar cells on part of the surface. Given reasonable improvements in thin film solar cells this figure could be 0.16 kg/kw for power production, not including other parts of the satellite.
Towards an Early Profitable PowerSat
http://space.alglobus.net/papers/SSI2010SSPpaper.pdf
Although purchasing one 12,500 kg per launch Falcon 9 costs $36.75 million, SpaceX representative Lauren Dreyer reports that packages of 1,000 launches can be purchased for $10 million apiece, which works out to $800/kg.
Launch costs are coming down with the Spacex Heavy to $1000/kg. But volume purchases can bring that down to about $500/kg.
Elon Musk is working to reusability to get launch costs to $10-100/kg.
====
There could be cases based on the size of the space based power system where it makes more sense to use 60% efficient thermal heat engines instead of solar cells.
Existing turbines are 10 kW/kg but can be far cheaper than solar cells.
Consider a PowerSat launched by a Falcon 9 assuming a mass of 100g/m^2, which at 45g/m^2 for the collection area leaves 2:6tons for all other systems. This leads to a square PowerSat 210 meters on a side. Assuming 8% sunlight-to-grid-power efficiency (20% solar cell and 40% transmission efficiency) this system would deliver roughly 5:28MW to the grid. A recent DOD report suggests that the U.S. military is willing to pay $1/kwh for power beamed to forward bases in Asia. Trucks transporting diesel can be ambushed, IR power beams cannot, and football-field sized receivers could fit on the larger bases. A 5MW system at this price would provide up to $46 million per year revenue, enough to pay for the launch in a little over a year. For commercial customers, the highest price this author could find world wide was $0.29 per kwh for industrial users in Italy in 2008. This could deliver up to $13.4 million per year { requiring a little over three years to pay for the launch.
large-scale storage is required to make solar power work at a large scale.
But, that storage could be very cheap. Daily variance can be handled by pumped storage and chemical batteries (or a myriad of other solutions, include molten salt).
Seasonal variance can be done cheaply: Just use excess power during the summer to electrolyze seawater into hydrogen which would be stored very cheaply underground (like NG) and burnt in NG type turbines - either combined cycle for efficiency or very cheap peaker-style.
Breaking water into hydrogen comes with an ENORMOUS loss. Flywheels would be a better solution.
Maybe a 40% loss. That's not a big deal in this context.
Overbuild wind and solar by 25%, use the excess 25% at 40% efficiency overall (for a net of 10% contribution to total kWhs), and you have more than enough to cover seasonal lulls.
Regarding flywheels: that's a solution for daily variation, not seasonal. It's capital cost is too high for seasonal - that's the same problem chemical batteries and pumped storage have.
Nick, as soon as you say, Overbuild wind and solar by 25% to accommodate seasonal variation, then away goes the validity of your prior statement:
Seasonal variance can be done cheaply: Just use excess power during the summer
Also it is far from clear to me that existing underground storage suitable for natural gas is also suitable for the tiny H2 molecule. For instance, to store the same amount of joules as natural gas either the pressure or the volume of the H2 storage must increase four fold. I think the better way to go is artificial synthesis of methane or liquid fuels.
That said, I agree with you that chemical energy burned in gas turbines is the best way to back up terrestrial solar seasonally, but don't expect it to be cheap.
away goes the validity of your prior statement: Seasonal variance can be done cheaply
Everything is relative. The US grid is 150% overbuilt, and those costs are accepted as part of the cost of doing business.
Also, keep in mind that this an extremely oversimplified scenario. In fact, those excess kWhs would probably be used far more cost effetively than in this scenario. They might be used to produce high value synthetic liquid fuels, and much of it would simply be shifted using DSM.
far from clear to me that existing underground storage suitable for natural gas is also suitable for the tiny H2 molecule.
It's being done now: http://en.wikipedia.org/wiki/Underground_hydrogen_storage
OTOH, as you note synthetic methane is a good alternative.
That's the thing. If this set of options were anywhere near as cheap as some of us think it is, it would already be being built. But it isn't.
It just hasn't been needed.
Why pay an extre penny per kWh for storage if it's not needed??
So your theory is that it will just tumble into existence in the near future, no problem, as soon as the cost structure shifts one more click?
That strikes me as a crazy assumption. If it were in that position, somebody very rich would be preparing it.
Storage does not become a severe problem until solar or wind becomes 40-50% of the energy source, which pushes the time horizon out quite a bit. With time, solar and wind can be just another evolution in the the parade of main energy sources: first wood, then coal, then oil, etc.
Nonsense. It's already a major problem in Denmark with just 20% wind. If you're in a not-very-windy location and is also not-very-sunny such as Germany, 20% is a major headache. It has to do with producing too much energy at times and most of the time too little. And you'd better have flexible natural gas for most of the other 80% of your electricity.
http://bravenewclimate.files.wordpress.com/2009/08/peter-lang-solar-real...
It's already a major problem in Denmark with just 20% wind.
That depends on your definition of "major problem". If you insist on using every last kWh then sure, even 20% will be a headache. Even very, very modest curtailment of peak wind production (less than 1% of wind-generated kWhs) makes wind power management much, much easier.
Your article by Peter Lang is badly biased.
"The paper takes the approach of looking at the limit position. That is, it looks at the cost of providing all the NEM’s electricity demand using only solar power for electricity generation. Looking at the limit position helps us to understand just how close to or far from being economic is solar power."
That's highly unrealistic. Trying to handle 100% of all power generation from just one source will produce absurd results.
A penny? Come on now. Hydrogen is very inefficient in lifecycle. It easily doubles your electricity cost. Chemical batteries are more efficient, but they don't come for 1 penny in the capital cost department. Not even 10 pennies per kWh. There was a devastating post on a "nation sized battery" which concluded the cost of just one week of primary energy stored in batteries is prohibitive. There's not even enough lead for it. And we're not even talking seasonal storage here.
You're way too optimistic here.
It won't beat coal. So China isn't veering and will continue to build a coal plant a week.
You need to read all of the comments above.
In short, using hydrogen for only 5% of generated kWhs, in very cheap ICE generators, wouldn't add much to overall system costs.
If you have a nuclear powered grid, then you could probably make do with that 5% storage.
With wind and solar, storage would dominate over generation. Just take a look at the production profile of wind & solar compared to the demand... you'll quickly produce too much power when its windy and sunny but most of the time will not have enough. Most of your kWhs will come from storage. It's not surprising that this is so, because of the low capacity factor of wind and solar, compared to the "capacity factor" of the demand (the grid).
If you want a closer look at how it all works, here's a more quantitative piece:
http://bravenewclimate.files.wordpress.com/2009/08/peter-lang-solar-real...
ICE generators running on hydrogen are only about 40% efficient. Electrolysis of water is 70% efficient. Compressor and other parasitic losses add at least 5%. That means you need 375% of the wind and solar generation just to cover losses. 3.75x the levelised cost of the wind and solar itself. So your storage kWhs will be prohibitively expensive (even without the ICE and hydrogen storage system cost). Don't underestimate the infrastructure cost of hydrogen. Underground gas field storage is cheap but you need a lot of hydrogen pipelines, compressors, valves and the like. These are much more expensive than natural gas compressors mostly due to the higher leak rate of hydrogen and its much much greater explosion hazard (as an industrial safet professional I know safety is expensive).
With wind and solar, storage would dominate over generation.
Not really. It would be far cheaper to use DSM and overbuilding.
Just take a look at the production profile of wind & solar compared to the demand... you'll quickly produce too much power when its windy and sunny but most of the time will not have enough.
If you overbuild wind & solar by perhaps 25%, then supply would be greater than demand perhaps 70% of the time.
Most of your kWhs will come from storage. It's not surprising that this is so, because of the low capacity factor of wind and solar, compared to the "capacity factor" of the demand (the grid).
The US grid's capacity factor is only about 35% right now, about the same as new wind power.
ICE generators running on hydrogen are only about 40% efficient. Electrolysis of water is 70% efficient. Compressor and other parasitic losses add at least 5%. That means you need 375% of the wind and solar generation just to cover losses.
No. Hydrogen backup would only be needed for about 5% of generation. That means that if it's only 25% efficient, you only need 20% more power overall. That fits nicely with our preliminary 25% overbuilding factor.
Underground gas field storage is cheap but you need a lot of hydrogen pipelines, compressors, valves and the like. These are much more expensive than natural gas compressors
I would assume colocation of generation and storage to minimize pipelines: electrical transmission is cheaper and easier. How much would you estimate for the compressors?
Why not use the WinFuels approach to convert surplus wind/solar power into distillate?
This consumes atm CO2, creates transport fuel, and converts surplus power too.
Regarding batteries, we at MPC have vetted this thoroghly, and our GridConPV1 project can produce
dispatchable PV power from an array in Ohio at $0.06/Kwh. Today.
INDY
Apparently, you haven't read Tom Murphy's other posts on the alleged cheapness of things like pumped storage and batteries. Your claims are not uncontroversial. Give those other posts a go and get back to us.
Oh, I've read them all, and commented on most: do a quick search for my name.
Tom was looking at storage for seasonal variations, but he was only analyzing solutions appropriate for daily variations.
Murphy dismissed the idea of chemical energy storage because of the efficiency losses:
But in a straight-up apples-to-apples comparison, the hydrogen method is a very lossy storage option.
That loss is indeed a problem for overnight, every night, use, but not seasonal and rare use. Chemical storage (H2, methane) works for those cases, and it does not required the extreme gigatons and gigatons mass of Murphy's battery.
Besides that power demand during day time is higher anyway (you don't need to store what you consume directly):


Lot's of energy is needed for water heating and cooling and heat energy can be stored very cheaply (my freezer and fridge stays cold all night and so does my insulated water tank - without electricity):
(just store your solar energy as low temperature heat energy - hot and cold)
And as long as fossil fuels are wasted in heating systems (where efficient heat pumps can be applied) there's no reason to store solar power in hydrogen, batteries and what not.
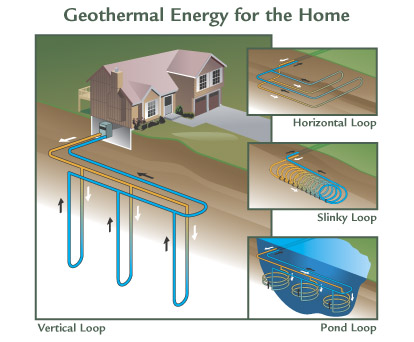
Simply use solar power to reduce fossil fuel consumption in the electric sector and thus store solar energy in the fossil fuels saved directly.
Excellent points.
Yes, daily/diurnal variation isn't that hard to deal with.
Wind and PV complement each other very well:
http://www.q-cells.com/uploads/tx_abdownloads/files/6CV.1.32_Gerlach2011...
And there's always more wind in the winter and more PV in the summer and don't forget the existing hydro capacity:
http://www.claverton-energy.com/european-hydro-capacity-compared-to-the-...
Besides: The fossil fuels saved can always be used for emergencies:
The gas power plants do already exist and can easily be operated at 5% capacity factor.
Good points. Ultimately it's won't be necessary to have anywhere near 100% grid backup, even for seasonal lulls.
Demand Side Management, geographic diversity, generation diversity and legacy fossil fuels (used just for occasional backup) will all have enormous contributions or usefulness.
Still, it's nice to have a good answer to Tom's very theoretical, very long-term concern.
I agree, you will never need 100% grid backup anyway.
But I don't even see any reason to discuss these hypothetical seasonal grid storage solutions.

Because there is simply and absolutely no market for it, as long as people can waste fossil fuels in heating systems and waste fossil fuels in inefficient pick-up trucks.
In addition: A hypothetical seasonal storage can in no way compete against a gas power plant at even 2% capacity factor. And this is your benchmark, because at this point fossil fuel use is really neglectable (compared to the 400% which is wasted now, when you include transportation and heating).
I would like you to build me a power station, I will be using it 5% of the time, probably at random intervals.
Now you just have to figure out how to finance & staff it; get back to me on a price /kwh.
I'm not saying it's a bad idea or impossible; just difficult in today's system.
Like everything else discussed on this site.
I would like you to build me a power station, I will be using it 5% of the time, probably at random intervals.
No Problem. In fact, it's already been done: they're called "peaker plants", and they're a major part of grid management right now.
You'd be surprised - some are used only 20 hours per year, but are still economic.
EIA has capital costs for Natural Gas electric plants at $.7k/kW. Nothing else comes close. As they can be (often are) located close to load, transmission build and loss can be low.
1. As Nick already said: Many peak power plants are already operated at 5% capacity factor.
In TODAY's system.
2. And the US already has 407 GW of gas power capacity: http://www.eia.gov/electricity/annual/pdf/tablees1.pdf
Which is already operated at only 20% average capacity factor in TODAY's system. And there's also 56 GW of oil power capacity at an even much lower capacity factor in TODAY's system.
3. Even if the peaker plant was expensive: It is irrelevant because you still get affordable electricity 95% of the time.
We already deal with varying electricity prices in TODAY's system.
Your food bill is also relatively low as long as you do not eat filet mignon with caviar every single day. What's not to get?
But let's just assume Today's system would not already exist. A simple gas turbine can be purchased for $0.2 /W and less (not combined cycle). http://www.generatormart.com/gas-turbine-generator-by-descending-kw-1.shtml

Natural gas is currently at $2.2 /MMBtu.
Let's say natural gas costs would be 6 times higher at $10 /MMBtu. At 38% gas turbine efficiency fuelcosts would only be 9 cents/kWh.
At 7% interest rate and 10 year amortization time the capital costs would be 6 cents/kWh.
Now add 1 cent/kWh for maintenance and you are at 16 cents/kWh. (Keep in mind this is a stationary gas turbine with an air filter (no birds, no hail, no ice, no water, no dust), no varying temperatures, low acceleration etc..)
At 6 times higher natural gas prices and only 5% capacity factor electricity costs are 16 cents/kWh - not even using an already existing gas turbine! And 95% of the time you do NOT need to pay this price!
This is why seasonal grid storage is pointless, unless it's already there anyway - see hydro power and biomass.
The assumption of many appears to be what to do when the natural gas is finally so depleted as to be uneconomic to recover. Some presume that's next year, I think closer to next century. In either case, the accurate description you lay out of the cost and scale of proven gas turbine technology argues for continuing to use it post natural gas with synthetically produced methane, or other synthetic chemical fuel (aka seasonal storage via chemical energy) produced from nuclear/hydro/wind/solar.
The point being: At 5% capacity factor and efficient use of electricity/energy fuel costs are simply irrelevant.
~Agreed. I do question the cost of maintaining a full up gas turbine fleet, in addition to the primary (future) sources of wind/solar/nuclear/hydro
1. You need less gas turbines. (Thanks to better interconnection, more demand response (heat pumps are flexible) etc.)
2. Gas turbines are maintained by the hours operated (time between overhaul). Lower capacity factor = lower maintenance.
3. We need to maintain many order of magnitude more vehicles with internal combustion engines now, than we will ever need to maintain gas turbines. One 300 MW gas turbine is much easier and cheaper to maintain than 1 million vehicles.
4. Even people in the future cannot simply live by banking each other and re-selling real estate to each other, some will always need to have an actual job.
My point was to try and bound the capital cost of a future electrical production system, versus the cost now. That's an important point in making a transition. Yes maintenance for low capacity factors is low, but it is far from zero. Structure oxidation, UV degradation, etc goes on, for instance, spinning or idle.
Point valid and worth making. But to quibble, I don't believe the kind of turbine you can buy for $.2/W will get 38% efficiency. It's more likely around 28%. Some reciprocating diesel gensets will do 38%, but that's on expensive diesel fuel.
Unless the market & technologies have moved significant since I looked a few years back?
Ok, but even if the peaker-plant would cost 25 cents/kWh instead of 16 cents/kWh it wouldn't change my point - since it's still only 5% capacity factor.
Besides: Even if this 5% capacity factor electricity would cost 250 cents/kWh: My 100% capacity factor electricity bill would still be ridiculous compared to my rent and my health care bill (since I'm using efficient appliances which will still be available in the future and probably even more efficient).
You can burn 85% natural gas in the 38% efficient recip diesel, for lower fuel and O&M costs.
Intermittency is easy to fix only if you don't care what the price of electricity is.
States that are now trying to legislate clean power, such as Texas (biggest electric power generator & user) and California (most aggressive RPS), are also wrestling unsuccessfully with how to both increase "clean" power generation and keep power costs down - without massive subsidies. Read about the current wrestling match this month in Texas:
"Texas Public Utility Commission willing to address looming electricity shortages by allowing wholesale prices to hit new highs during peak demands for power."
We can't fix our growing energy problems with windmills and ground solar. The cost of fixing their unreliability is too high and too limiting going forward on the massive scale required. Space Solar Power is the best LARGE scale, low CO2, dispatchable, safe, clean, reliable and low cost power generation technology, which is why the Japanese and Chinese are putting many Billions into SSP while the US bleeds aerospace technology and spends almost nothing on SSP.
Space Solar Power Institute
"We can't fix our growing energy problems with windmills and ground solar. The cost of fixing their unreliability is too high and too limiting going forward on the massive scale required."
On your say-so?
It's "..the best LARGE scale, low CO2, dispatchable, safe, clean, reliable and low cost power generation technology.." How can you presume to say such things?
SSP is not the best, is not, in fact ANYTHING.. until it actually exists and shows that it holds and that we can maintain it. You think grid storage and intermittency is too hard, but keeping a fleet of GEO spacecraft on a reliable flying and delivery/work schedule is both feasible and reasonable?
'To Infinity, and Beyond!'
Thanks for the comment. Perhaps you don't know that we have been keeping a fleet of thousands of satellites in orbit for many years. The concept works pretty well.
Yeah, communications satellites are a little more complex than windmills, but they provide far more reliable (24/7) service than windmills ... and plenty of excellent jobs.
I claim SSP would be "the best LARGE scale, low CO2, dispatchable, safe, clean, reliable and low cost power generation technology" because that is my opinion after having studied and participated in the work of many researchers in the technical fields necessary for SSP for decades. And also having done strategic planning and advanced development for many years for a large electric power company.
SSP's component technologies have been very actively researched for many years and so I encourage people to examine SSP more carefully before laughing too hard, until they can identify and demonstrate a better solution for our energy/economic/environmental challenges. The global/US outlook on that score is pretty gloomy currently; it is better to light a candle than curse the darkness.
Competition among solutions is a good thing, unless you are among the folks that prefer to watch and wait and buy their SSP from Japan or China.
I claim SSP would be "the best LARGE scale, low CO2, dispatchable, safe, clean, reliable and low cost power generation technology"
Great claim. What are your facts backing up this claim?
because that is my opinion
So you have no actual facts to share.
Carry on then.
Darel;
A generation of comparatively TINY comm satellites is not going to prove the concept of power satellites. The physical dimensions and the work required to test, prove and implement this has differences that can hardly be guaranteed to the degree you have been stating them.
Moreover, a Comm Satellite is paid for with the value of Concentrated Long Distance Communications, which can command prices that operate entirely differently than those of the energy market, which will be the yoke and chain around SSP. Until you can show the quantity of power produced against the CapEx and Operating Costs, the actual life expectancy of Operating Systems, and the Price paid for delivered Power.. then you are really still only talking about vaporware.
How in the world are we supposed to accept the promises people are willing to offer for this complex, large and barely accessible technology?
Jokuhl, what do you need beyond communication satellites to show that the concept of power satellites makes sense?
Both are (or will be) in GEO.
Communication satellites last for decades. What reason(s) do you have that power sats will not last at least that long? (Communication satellites usually run out of station keeping fuel before other parts fail. Resupply and maintenance should be assumed with power sats.)
Both send power to the earth by microwaves. Power sats send a lot more, but the historical analogy is that telegraph lines showed that electrical power transmission was possible and by about the same ratio.
Power sats are a lot bigger. That generates some scaling issues, but those are well understood in the dynamic models.
The key, of course, is low cost transportation but if you have a few GW in orbit, propulsion lasers and the very high exhaust velocity they are expected to have reduces the cost to well under $100/kg.
Re prices, it is called design-to-cost. If the physics/engineering/economics model will not come in at half the price of coal or less, it's not worth doing power sats. Why? Because the front end investment is large enough that you need to take substantial market share away from higher price power sources.
Also, if you can't project this into displacing fossil fuels in a decade or so, it may too little, too late.
If you do have a cogent reason power sats won't work, please don't keep it to yourself.
The argument that existing microwave communication links from GEO to Earth in any way offer proof-of-concept for power transmission is grossly off. A few tens of Watts of microwave power can be beamed to bathe the entire Earth and be called a strong signal to receivers on the ground. Now up the power by eight or nine orders of magnitude and simultaneously narrow the beam footprint by 6 or 7 orders of magnitude and you've got a horse of a different color. The fact that electromagnetic waves can travel from one place to the other is not disputed.
You are a bit out of date.
"The Spacebus 4000C1 has a height of 4 metres (13 ft), and is capable of generating 8.5 kilowatts (11.4 hp) of electricity. The only C1 to have been ordered so far is Koreasat 5 for Korea Telecom of South Korea. It was launched by a Sea Launch Zenit-3SL from the Ocean Odyssey platform on the equator, at 03:27 GMT on 22 August 2006." http://en.wikipedia.org/wiki/Spacebus
http://en.wikipedia.org/wiki/Alphabus satellite is projected for 12-18 kW with growth to 22 kW.
As for narrowing the beam, that's already been done with big phased array radars.
It's just optics and the math and physics behind the design is over 200 years old.
Scaling up the propulsion lasers worries me more, but lasers pumped with laser diodes have already been scaled up from under a mW to 100 kW over about the last ten years. That's at least 8 orders of magnitude. Going from 100kW to 1 GW is only 4 orders of magnitude.
Mind you, it's *NOT* going to be cheap. Dr. Jordan Kare makes as case that lasers in huge numbers are going to cost around $10/W.
As for narrowing the beam, that's already been done with big phased array radars. ... Mind you, it's *NOT* going to be cheap
Sound approach. Test the things particular to space ... in space and the remainder on the ground. For instance, test cost per kg to launch, test Murphy's reliability contentions:
Panels in space will be subjected to a much harsher cosmic ray (and damaging debris) environment than those on the ground, so we should reduce the lifetime to, say, 20 years.
and
The [ground solar] installations ... will last longer.
"Panels in space will be subjected to a much harsher cosmic ray (and damaging debris) environment"
Radiation is one of the reasons I tend to favor thermal engines. But for physical damage, consider a hailstorm.
Another reason to favor turbines is the flash over problem. Hard vacuum is a heck of a good insulator, but that's not true of the plasma you get from even a tiny flake of cosmic dust hitting at many km/s. Turbines are relatively compact objects and you can put the connections to the microwave generators in pressurized or at least shielded pipes. 10 GW (what you need in space for 5 GW on the ground) is a lot of volts and amps no matter how you slice it.
But for physical damage, consider a hailstorm.
Right, those one-off incidents are one reason why it is not obvious to me that ground based solar would be far more reliable than space based. Another is that I doubt the one-off incidents are the primary failure mode on the ground. Instead, I guess that daily mechanical expansion-contraction cycles and the stress they place on structures and electrical connections are the larger culprit. LEO satellites also see severe daily temp cycles, but a solar pointed GEO satellite could have a ridiculously stable temperature most all the time. I'd like to see that advantage up against, say, the radiation damage disadvantage.
Instead, I guess that daily mechanical expansion-contraction cycles and the stress they place on structures and electrical connections are the larger culprit.
PV on existing building reduces the load on the grid, since the building can cover some or even all of its demand directly from the roof. (In fact: the Swiss hydro power plant operators are already crying about the German PV plants, because on sunny days they take their lucrative midday peak: http://www.drs.ch/www/de/drs/nachrichten/wirtschaft/301909.schattenseite... )
PV modules have a 25 year warranty: http://www.sunelec.com/Specs/feb2012sale/STP205-18.pdf
And can be purchased for less than $1 /W: http://www.sunelec.com/
So, even if it needed to be replaced after 26 years - that $1 will have produced over 40 kWh - so what?
That's all another issue. What is the reliability compared to space?
The module price in a distant factory increasingly irrelevant. The installed price must be paid. Otherwise, with a SBS array it could be built on the ground and declared finished, never mind those launch costs.
What is the reliability compared to space?
The PV-modules on the ground have been tested and are warranted 25 years.
The module price in a distant factory increasingly irrelevant.
Besides that sunelec is a US distributor and besides that the cheapest PV-modules are still being produced in the US (First Solar).
This container ship gets over 600 mpg per ton: http://en.wikipedia.org/wiki/Emma_M%C3%A6rsk
A PV-module has more than 10 W/kg. So with one gallon of fuel one can ship 10 kW over a distance of 600 miles.
And let's say one barrel of oil/fuel costs whooping $500, this would add only 0.016 cents/W to the costs of the PV-modules per 600 miles traveled.
But for physical damage, consider a hailstorm.
And one can remove the panels, tilt to a low-profile for the hail, or even cover.
In all humility, my objection is entirely Heuristic. I'm sure these research teams have piles of accurate calculations and analyses... but what are the underlying contextual expectations that these are built on, since THAT is what holds up a big, expensive endeavour in the end.
..so when you say, "Power sats are a lot bigger. That generates some scaling issues," I find that the casual shrug towards this scaling question is not likely to be as benign a problem as you seem to make it.
I can't prove this.. it's just a pattern I'm watching in other big endeavours that become simply more ungainly and brittle as they grow, not in fact more facile and durable. In many ways, I think the Biggest Wind-power systems will face similar problems with resilience, as an industry, if not Turbine to Turbine.
Another promise this issue reminds me of is the expectation of Bush/Rumsfeld etc, that their wars would take a few days, and Iraqi oil would largely pay for the endeavor.. barely anyone would even die. Is it unfair to associate that big, predictable disaster with this one? Probably not.. so far, to me, it has only quacked like a duck. I have said, 'That still sounds like a duck.. but I will take a look when there is something to see.. and maybe find out that I was off by a bit..'
There are many processes down here on Earth which get more affordable as they scale up.. and others, like many of the current Reactor Builds and License-Extension Upgrades (Finland and Point Lepreau,NB for example.. ) that are taking place, that seem to be going rapidly the other way.
We often have to make choices and start to work up our judgements with incomplete information .. because it might be far too late to jump wisely, once the hard data is in and nicely charted. (EDIT: By which I mean the Actuals, not the Blueprints)
Already, our power sources are owned by too much big money, and they are generally far removed from where we live and work. From a purely philosophical/design perspective, this scheme is simply heading way farther down that same bad road, leaving us all heavily dependent upon long, complex umbilicals owned and controlled by Billionaires who show less and less interest in our common DNA.
We need to get our power back.
I don't share your big concern about the locations of the power & control of the transmission. But I do completely agree about the scaling of complex endeavors.
As for Bush/Cheney/Rummy, I don't think those guys believed their own bullshit even at the time. Their optimistic sales pitch on the outcome of that invasion wouldn't have fooled me when I was 10 or 12 years old. And not because I was anything special.
Peak prices aren't the same as average prices.
Renewable power will be cheap at during peak production, expensive during production lulls, and on average very affordable.
Actually it's very easy, because Wind and PV complement each other very well:
http://www.q-cells.com/uploads/tx_abdownloads/files/6CV.1.32_Gerlach2011...
And interconnected wind farms provide baseload:
http://www.stanford.edu/group/efmh/winds/aj07_jamc.pdf
And the existing hydro storage is already enough to cover Europe's entire electricity demand for 22 days:
http://www.claverton-energy.com/european-hydro-capacity-compared-to-the-...
There are no nights and no dead calm periods which last that long!
But most importantly you don't even need a 100% renewable grid anyway, because you simply need to reduce the fossil fuels wasted in heating and hot water application and replace them with heat pumps and reduce pick-up truck use in transportation. In that case you can easily run your electric grid with 50% fossil fuels and still reduce fossil fuel consumption by over 90%:

Spain was already at 21.7% wind power in February and Spain is not even well connected to France which also explains the huge electricity price difference:
http://www.earthtechling.com/2012/03/spain-blows-away-monthly-wind-energ...
The big problem in both of those states is the market structure after de-regulation.
1. People in the not too distant future may have to get used to the idea of intermittent power. Power might go down over night for some applications via a lockout feature in smart appliances. No electric clothes driers after 10 P.M. for example. Food refrigeration would be given a higher priority whereas air conditioning would be subject to limitations.
2. The cost of high efficiency PV might be greatly reduced by a new process that "exfoliates" wafers using proton implantation. Very little waste compared to sawing them off the boule. It could factor in as a reduction in mass, but likely not significant as it would make up a small fraction of a space born panel.
3. Let's not forget flywheels as a storage medium. Very efficient, not difficult to build, repairable, scalable, available now.
4. In a low-energy per capita future, large scale space projects may be out of the question.
The first satellite program I worked on was Insat for the government of India. Putting two satellites in orbit with multiple ground stations turned out to be much cheaper than running land lines all over the Indian subcontinent. In this case it was information beamed back to Earth, not gigawatts of power. Harvesting energy and materials from space will likely never be worth the costs.
Hey, I'm already used to intermittent. I myself am very intermittent. I do pretty near nothing all night. And as for solar here on earth, simple, use a heat engine instead of PV. Of course I myself prefer stirlings, which are my game, but there are plenty of other thermal engines for bigger sizes.
I put 1kW of PV on my house just recently. Great stuff!, But here where I live, a typical site in USA, there are plenty of cloudy days, not to mention nights. I did the numbers, and if I had a stirling using the same area as the PV, and used it only during bright sun, and other times burning wood (stored solar), I would get lots more watts/ $.
And forget all that sobbing about how expensive stirling space engines are. The exact same concept could be FAR cheaper if made by a commercial manufacturer instead of those one-off cost-no-object things for space.
Another thing. Tom Murphy once made a remark I share- PV is great but I worry that I myself would have a hell of a time making one in time of need. Stirlings and other heat engines can work using only 19th century iron banging, so in that low energy future, I go for the iron--in my back yard, not in orbit.
And forget all that sobbing about how expensive stirling space engines are.
http://volodesigns-sterlingproject.blogspot.com/
FAR cheaper if made by a commercial manufacturer
http://web.archive.org/web/20010428154616/http://omachron.com/papers.html
http://forums.segwaychat.com/archive/index.php/t-34.html
Tom, that is a very good analysis of power satellites.
The solution to the economic problem as the NASA study pointed out is to reduce the cost to GEO to $100-200 per kg. At $100/kg, an installed watt cost about $1.60 based on five kg/kW, $900/kW for the parts and $200/kW for the rectenna. That’s low enough to make synthetic fuels for a dollar a gallon on off peak power.
The problem, as you point out, is the low payload fraction to GEO using conventional chemical rockets. You have to give up on chemical rockets and go to heated hydrogen to get the performance you need. (See http://en.wikipedia.org/wiki/Nuclear_thermal_rocket for performance data on heated hydrogen.)
But something that only recently occurred to me is that if you are building hundreds of GW of power satellites, then it’s easy to use the power from the first one for big propulsion lasers. I have run the calculation. Start with a Skylon (Reaction Engines’ design) derived vehicle that burns air and hydrogen until it runs out of air at 26 km and Mach 5.5. Then it switches to 2 GW of laser to heat hydrogen from Mach 5.5 to orbital speed. It will put 20 tons in GEO three times an hour or half a million tons per year. That’s enough to build 100 GW of new power sats per year.
The energy payback time (a more useful measurement than EROEI for renewables) is about two months. A previous version of this scheme is on The Oil Drum. http://www.theoildrum.com/node/7898
The problem is to get a 500 MW seed propulsion laser to GEO. That’s expensive, the laser at $10/watt is $5 B and the transport to GEO using Falcon Heavy is perhaps $20 B. The rest of the 2 GW ($15 B more for lasers, $1.5 B for transport) is brought up in 1/4-scale vehicles. The income from the completed transport system is about $50 B a year and the gross margin is at least $25 B, making the payback time less than three years.
If one part in ten of the new power sats was fed back into more laser propulsion capacity for a couple of years, the transport rate to GEO would go up to 5 million tons a year, enough for a TW of new power per year. With slightly more growth, the entire world could be off fossil fuels in less than a decade.
If you want to see the spreadsheets, ask.
The physical world is way more complicated than a spreadsheet, Keith. Isn't it true that "get[ting] a 500 MW seed propulsion laser to GEO" is more than just expensive? It has never been done, right?
One suspects many more such practical flaws dwell in your spreadsheets...
"It has never been done, right?"
There are vastly more things that have not been done than have been. I think the human race is in big trouble if we are limited to the known solution space for getting out the energy/carbon mess.
Call us when there's a cheap working seed laser propulsion rocket. In fact, call us when there's one that works at any cost.
"cheap working seed laser"
I said a 2 GW laser would cost ~$20 B and about the same amount to put a 500 MW seed in orbit.
If you want to see a working 105 kW laser, see slides 13, 14 and 15 here:
https://docs.google.com/file/d/0Bzm7IoVgps00ZWw0eng4TmRSWkdNWFhpZFk2QzNQ...
Power projects are huge, http://en.wikipedia.org/wiki/Three_Gorges_Dam so this isn't far out of line.
And $75 B is what has been spent on the F-22 fighter.
I grant you though that an energy collapse and die back to 1-2 B people in famines and resource wars is more likely than solving the energy and carbon problems in any way.
we are limited to the known solution space for getting out the energy/carbon mess.
The 'known solution space' of private interests getting paid with public money is why 70% of the money spent on Carbon control doesn't actually control Carbon.
Why should the 99% sign up to further enrich the 1%?
And the addition of the ammount of solar energy that formerly did not impinge upon the earth that these satellites will collect and beam to earth, eventually mostly becoming heat in the atmosphere... Will have what effect?
Arthur C. Clarke discussed this - paint the roofs white (which we should be doing anyway).
I don't know if the numbers are in the correct magnitude.
In the past discussions I asked that exact question and got a whole lotta handwaving. Its just like the "we'll use Hydrogen" solutions - the question "what is the long term effect of widespread H2 gas in the biosphere" needs to be answered.
You have to give up on chemical rockets and go to heated hydrogen to get the performance you need.
And no mention of the effect of the effect on that Hydrogen on the atmosphere of the Earth.
Placing Hydrogen in the Ozone layer will not be helpful to the Ozone layer, will it?
If I understood you correctly, a 30m dish would transmit about 2 GW?
Given the conversion-inefficiencies in space and the waste-heat generated with it, how would this several 100 MW waste-heat be dissipated?
Fantastic question. I did not consider this facet. I did not explore the efficiency of conversion into microwaves, but even a 1% loss is a big deal! A standard dish wouldn't work at all, with a compact generator and a passive (parabolic-type) reflector. It would have to be a distributed phased array of antennas, and yes--probably spread out to rather larger dimensions than 30 meters. It is tempting to think of the transmitter elements spread throughout the solar panel array, making for a very large footprint and good beam-forming potential. But at higher frequencies, the formation-flying requirements (or metrology) to correctly phase the signals to the millimeter level would present challenges: especially for these giant "sails." Lower frequencies simply mean bigger antennas again.
Again from the Al Globus article Towards an Early Profitable PowerSat
The product of the sending and receiving system minimum diameters is linearly dependent on wavelength. There are narrow transmission windows in the atmosphere at around 1 to 2 micron which, if exploited, could reduce the minimum antenna diameter product by a factor of around 60,000-120,000 at the cost of some efficiency. At 1 micron this would permit a five meter on-orbit beam transmitting to a minimum 32 meter receiver on the ground. This enables small PowerSats, at the cost of much higher beam density and associated problems. Assuming the best conversion efficiencies demonstrated in the lab, for a 5MW-to-the-grid facility with a beam at 10x the power of sunlight the receiver must be about 45m in diameter. Also, as the near 1 micron windows do not survive cloudy conditions, such PowerSats may be most suitable for desert-like locations, where, fortunately, there are substantial electrical power markets such as Los Angeles, San Diego, Las Vegas, parts of Australia and North Africa.
Infra-red power beaming has been demonstrated by, among others, LaserMotive, which won first prize in a NASA sponsored power beaming competition. This involved a vehicle climbing a one km tether using power beamed from the ground. LaserMotive delivered 500w at 0.808 micron with around 10% efficiency over one km using o -the-shelf lasers, custom optics and custom solar cells. High efficiency was not essential to the project. The LaserMotive chief scientist suggests that 25% could be achieved today and perhaps 40% with near-term technology
Alfalight, Inc., a diode laser manufacturer, recently demonstrated 65% power
conversion efficiency as part of the DARPA Super High Efficiency Diode Sources program. This program has a goal of 80% efficiency. As lasers produce energy at essentially one wavelength, it should be possible to develop high efficiency 'solar' cells to convert this laser light back to electricity. The low efficiency of conventional solar cells is in part due to the difficulty of capturing energy at many wavelengths. Spire Semiconductor LLC produced a concentrator photovoltaic solar cell measured by the U.S. Department of Energy's National Renewable Energy Laboratory (NREL) at 42% peak efficiency. These results also suggest that something near 40% end-to-end efficiency may be achievable. While this is less than the 63% estimated for microwaves, massive reduction in system size provides ample compensation.
The question wasn't about the size of the antenna but about dissipating heat in space (one antenna with 2 GW and conversion inefficiencies). Since this wouldn't be an option:

Thank you Tom
Precise and too the point, I fail too understand why all these Techno - narcissists come up with these expensive extremely complicated ideas. Complication only leads to breakdown. I can remember many years ago reading an article about NASA spending millions developing I think it was a ball point pen that could write in space. What did the Russians do they gave there Cosmonauts a pencil, problem solved. Make it simple make it cheap. The cheaper Solar panels become the sooner these silly idea will disappear. The only problem as I can see with this pie in the sky idea solves is intermitancy and as far as I am concerned. Nobody seems to ask can we live with it. The Dutch built an empire on intermittent power, when the wind didn't blow they didn't grind corn or pump water. When it did the miller worked all the hours god sent, taken over a year they still ground the same amount of corn as the year before, it wasn't real a problem.
I personally think that this problem is on the way to being solved. You might want to take a look at this firm in England they seem to have the right Idea. No fancy batteries no toxic chemicals, simple and cheap easily manufacture with the manufacturing base we have now so no new industries have to be built which means that it can be built quickly if necessary.
http://www.isentropic.co.uk/
You might want to comment on it in another article
York, I would be the first to agree with your general note that complication leads to breakdown. Auto navigation systems, websites, operating systems, air bags, hell, even electric windows-- all over-engineered junk, IMHO.
However, the anecdote about the space pen vs. pencil is a myth:
http://www.snopes.com/business/genius/spacepen.asp
The Fisher Space Pen is, IMHO, a marvel of engineering. I just bought another one online for $24 with shipping, which is how I happen to be up to date on this bit of trivia. Cost about a million private-sector dollars to develop, and worth every dime.
Working in community mental health, working with high-risk clients and writing on clipboards? I need a pen that won't fail and will write at any angle, and nothing else works as well.
For a high-end ballpoint for special occasions, sure the Faber Castell is a great instrument, nice weight, writes beautifully. If you need something in a hurry, Zebras aren't bad. I have two Tornado 1957's that look really cool, but the ink bleeds and they don't feel right on the page. Montblanc's are junk; my mother had one that clogged all the time, and you lose it, you're out a lot of coin.
For day to day reliability and good feel for the page? Give me the Fisher space pen every time. Perfect doomer pen!
The longer it stores the temperature difference, the lower the efficiency because heat will flow in and out of those insulated tanks.
I am skeptical about the claimed efficiency. It might be the peak efficiency when it has the greatest temperature difference to power the generator.
"about dissipating heat in space"
Indeed, getting rid of waste heat in space is a pain in the ass. It takes a square km to get rid of 500 MW of waste heat at reasonable temperature. At an emissivity of .95 and a temperature of about -12 C the radiation is 250 watts per square meter (ignoring back radiation from the dark of space). How to do this at low mass has been discussed here before. Eric Drexler figured it out in the late 70s.
I can post a graph out of an excel sheet if someone can point me to how you do that on the oil drum.
And when you're close to a star, like the Sun, you have to orient the radiating surfaces facing north or south to avoid heat from the star. In GEO each side of the space craft gets solar exposure during the day. Only the north/south panels face deep space. Now consider the maximum diameter of a fairing is about 5 meters on a heavy lift launch vehicle. Rockets will have to get a lot bigger or some assembly in LEO will be required. That gets us into the orbiting factory problem real quick.
Then we have to know how many slots such an array of satellites would require and conversely how many existing satellites would have to be parked or de-orbited. And how close can they be spaced? Remember that transfer orbits may bring satellites uncomfortably close to each other.
"assembly in LEO will be required"
Sorry, Boeing wanted to to this back in the 70s and "did the math." Even in those days the space junk problem made junk out of a satellite self powering from LEO to GEO. So cargo has to go the GEO and the power sats have to be assembled there.
Power sats and comn sats coexist just fine.
The infrared windows are useless in clouds. I've spent many a night sitting under clouds at Palomar wishing I could do my 2 micron observations through clouds.
In truth, my 100 GHz is still too high frequency. Likely want to operate under 10 GHz before worries of clouds are gone.
It is indicated in the paragraphs that I quoted
PowerSats may be most suitable for desert-like locations, where, fortunately, there are substantial electrical power markets such as Los Angeles, San Diego, Las Vegas, parts of Australia and North Africa.
Because of the cloud factor. Alternatively if there are several satellites and several ground stations then the satellites can beam to ground stations that are clear.
Also, Al Globus had an updated paper
http://space.alglobus.net/papers/TowardsAnEarlyProfitablePowerSatPartII.pdf
"Towards an Early Profitable PowerSat, Part II" Al Globus, Ion Bararu, and Mihai Radu Popescu, International Space Development Conference 2011, National Space Society, Huntsville, Alabama, 18-22 May, 2011. This paper suggests an approach to developing a small, single-launch SSP system that may be profitable in certain high-priced niche markets. The underlying technologies could be developed by a modest R&D program, suggesting that critics who claim SSP is multiple orders of magnitude from profitability are wrong.
http://www.currentresults.com/Weather/US/average-annual-sunshine-by-city...
85% of the time sunny in Las Vegas, Nevada
210 Clear Days is the average number of days annually when cloud covers at most 30 percent of the sky during daylight hours
73%, Los Angeles, California, 147 clear days
85% sunny, Phoenix, Arizona, 211 clear days
78% sunny, Sacramento, California, 188 clear days
66% sunny, San Francisco (bay area), California, 160 clear days
It would be a cruel twist to invest so much in an elaborate space-based power system only to find that it's really only useful in the places where ground-based solar excels. Not sold on anything bu microwave. And even that seems wretchedly complex (thus unlikely).
So there are plans proposed by Al Globus to achieve results with a few hundred million. There is already a megawatt(s) or so of space solar power for satellites in space.
Japan is willing to spend a few billion on it.
How does this compare with nuclear fusion. Nuclear fusion has had decades of funding around the billion dollar per year level (all nations added up). No commercial power generation yet.
I support nuclear fusion research and space based solar power research.
Energy infrastructure is a multi-trillion per year effort.
If you disqualify space based solar power because of it being 4 times more expensive based on a first order and wrong analysis then you should not have ground based solar because it is still over 4 times more expensive than coal and nuclear power.
Get space based solar for expanded space based efforts and for on the ground niches and let it grow. Hopefully to the 500 MW level to potentially reduce costs of space launch per the Henson plan and other applications.
Countries that did not fund developments of the Americas several hundred years ago missed out and were weaker than those that did.
you should not have ground based solar because it is still over 4 times more expensive than coal and nuclear power.
What is the cost per watt for Nuclear Fission for TEPCO on March 10th 2011?
How about the cost per watt for TEPCO now?
Do show what the cost per watt really means for Nuclear Fission.
Yes, I think this is what even the small, <1%, eclipse time at GEO is a major problem. The satellite would otherwise have a steady state temperature but experience quite a thermal shock going in / out of shadow.
Not as bad as you might think. Such a satellite would have a tremendous thermal load and a lot of mass. The big problem is still getting rid of the waste heat. Moving from light to shadow in GEO can create stray voltages on the chassis. I would imagine a larger chassis would have to deal with larger voltages?
I don't think thermal mass helps much here, as in it would in, say, some kind of low area to volume ratio cylindrical communications satellite. A solar array wants to maximize the area to volume ratio, i.e. any extra thermal sink/source not in sun exposure is expensive overhead.
This all guess work from a lot of ground based systems thermal management experience, none in space:
Consider just one PV module/panel to start. In sunlight it's receiving 1366W/m^2 and has mass on the order of 1kg (give or take depending on what you believe in the discussion above). It hopefully converts 20%. The rest is absorbed/radiated away to reach an equilibrium temperature. Radiator mass comes at a premium at GEO, so I assume some high-as-possible temperature for efficient PN junction operation is designed for, maybe 20C? Then comes the shadow. At onset the radiator is still radiating away maybe 500W at 20C (absent a disconnect scheme). If the specific heat of the panel is ~0.5 J/gm/K, then the 1kg panel's temperature drops (initially) ~1 degK/sec. The rate falls immediately of course but then shadow it falls for 70 minutes.
All of the controls, telemetry, command, and critical payload controls would have battery back-up including heaters to maintain voltage in the batteries. This is common to all GEO satellites. The boxes I've been involved with run at room temperature, more or less. They're spec'd to go much colder and warmer, but that's just in case of a mess up somewhere. Junction temperature in the semiconductors is always quite cool. Maybe 50C max?
BTW, all the better com satellites are three-axis stabilized, not spinners.
PN junction efficiency is temperature dependent:
Temperature coefficient depends on the chemistry of the solar cell. Cheap polycrystalline silicon has a tempco of 0.5% per degree C.
"then the 1kg panel's temperature drops (initially) ~1 degK/sec."
You put your finger on one of the problems that has to be solved. I have suggested making the structure out of rolls of Invar foil run through a roll former, like they make no-splice gutters with. Others have mentioned that carbon fiber has thermal expansion as low as Invar.
You also have to be sure that the bending oscilation doesn't have a period that is a multiple of 24 hours to keep the bending from building up.
I am inclined toward thermal cycle designs because they are smaller but the progress in PV has been impressive.
The article provides a good analysis, but unfortunately it uses a pretty ridiculous design.
The idea of launching large areas of solar cells is laughable.
Extremely lightweight mirrors have been demonstrated in space, and given that launch costs are (mostly) a function of weight, it is clear that any space-based power gathering system should be mostly lightweight mirrors.
Also, given the 50% end-to-end efficiencies of a microwave link, surely direct re-transmission/reflection of the sunlight is the way to go with approximately 75% efficiency.
Sunlight has a +-0.005 divergence, at 36,000km that is +-180km a very big spot on the earth.
I'd imagine one wouldn't use a flat mirror. I don't know about the effects of the atmosphere but it's possible one could use active optics similar to astronomy.
Clouds would clearly be an issue...
Are you thinking (A) "shine the light on the earth & convert it to PV electricity" or (B)"Concentrate the light in space onto PV panels?"
"A" sounds even hotter than microwave transmission; that amount of light and heat would be discouraged anywhere near civilization (or astronomy!). But the entire system would be much simpler. Also since it would be guided anyway the military would go for it as a death ray.
"B" would drastically reduce the orbital mass; but how much concentration can you have before you have cooling issues (as discussed)?
As long as we're blue sky engineering...
Deploy large mirrors in orbit. Use them to pump a huge laser tube. Point the beam wherever you want the energy... Or any place that you don't like the looks of.
Very nice essay.
To construct my own solar-power system I ordered some panels which were shipped on pallet via container ship, and subsequently bolted to my roof with off-the-shelf hardware, and hooked to a bank of golf cart batteries. If a meteor or some pigeon poop hits one of the panels, I'll get out a 10-foot ladder and do a "sky walk" to my nearly-flat roof to repair or replace it. When my inverter blew, all it took to replace it was a screwdriver and some profanity.
If I had to get it into geosynch orbit, the odds are it would still be on my "to do" list. The various thresholds of do-ability were quite achievable.
Contrast this with the actual cost & problems of the solar array on the International space station, in low earth orbit. Then make it more difficult by putting it into geosynch.
A whole bunch of other problems come up. First, you need to get launch costs to LEO and geosynch down by a huge factor, which doesn't seem to be happening. Then you need to figure out how to fund it in the teeth of a "greater depression". You need to sell it politically, since the idea of the USA having enormous microwave-shooting structures in the sky is a proposition which would not be received warmly by other nations, and it would be a lot cheaper to frag them than build and deploy them. Etc.
Previously on this site, I've said that space solar power is a useful litmus test for systems blindness. The real-world constraints imposed by considerations of aggregate probability are telling. It's another sort of "doing the math": the odds of developing a radically cheaper and more reliable heavy-lift capability to orbit, times the odds of achieving radically cheaper ways to get to geosynch, times the odds of getting financing, times the odds of it being politically do-able, times the odds of every other necessary breakthrough, etc.
Space stuff is very cool. But it's pretty likely that the last human to make it to orbit - ever - has already been born. We're just beginning the long permanent decline of easy energy, and it'll be a rough re-entry.
Oh they'll warm up to it alright if we point it at them...
Sorry, too juicy to resist.
Heh. It was a conscious choice of words... I figure if you can go to the trouble of writing the essay, the least I can do is offer a good straight line...
... and you are doing a service. I've actually seen philanthropists pull large funding from very useful species conservation work and redirect it into space solar power because they have been assured that the numbers add up. That's a real-world consequence. Nate Hagens, formerly of this site, talked of a "tragedy of the energy investing commons" which would occur when people start to realize that oil and easy energy are going away, and start throwing money around wildly.
To qualify this, as I did in the past when I've commented: I was inspired by Gerard O'Neill back in the early '70's and have been a passionate space enthusiast all my life. I've had a lot of interaction with NASA folks and astronauts (and scifi writers) in some of my projects; it would be hard to find a bigger fan of space exploration. But heavy-lift space programs are winding down, not up. And there are a lot of ladders and empty roofs available...
cheers...
For a whole list of reasons, the microwave transmission is a really poor weapon.
However, the propulsion lasers are a different kettle of fish. One second exposure from a 2 GW one will kill a person by burning off the top foot.
I used to be concerned about this, but it isn't any different from being hit will a Hellfire missile dropped from a Predator drone. The US can already kill anyone, including our own citizens, anywhere in the world. So how do the propulsion lasers make this worse?
However, if the US had access to propulsion lasers we could chose to ignore upstart countries that are trying to make nukes. And for certain there would be no reason to fight a ground war anywhere in the mid east.
???? I'm having a hard time forming this image in my head - but would a tinfoil hat help?
"would a tinfoil hat help?"
Taking you seriously, not much. (Hats are really useful for microwaves.)
If the laser beam came straight down and the hat totally reflected the laser, you would still be fried by the ground turning white hot all around you.
Long ago, I made a three-foot diameter mirror from an X-band radar dish. It concentrated its one kilowatt of sunshine down to about a half-inch diameter hot spot in the middle of a bunch of aberrations. A fun demonstration was to try and reflect this using an ordinary pocket mirror. The backing would flash off and the glass would break. Shiny aluminum soda cans would ignite with an audible "pop" when held in the focus.
Right on, greenish. A little PV on the roof or in the back yard + a little storage + a good dose of negawatts + some negajoules + a bit of adapting/flexing to natural cycles; these don't seem to be the sort of things industrial citizens want to invest in. Space-based power for a spaced-out populace. That'll do it :-0
Our collective delusions of grandeur are what got us into this mess...
Thanks. 24/7 baseload power is certainly an indulgence for most things, since a human body tolerates hiatus just fine in many ways. No need to store all the power that comes in, I just use most of the solar generated during the daytime with the batteries acting as a buffer, I never deep-discharged them. Running a pool pump, running freezers on timers with enough thermal mass that they don't need to run at night, running fans, etc. Doesn't take much power for LED lighting or powering the computers in the evening, etc. We have greatly exaggerated notions of what we "need". It's a heckuva lot easier to scale down silly wastes of power than it is to hang large devices in geosynch orbit, and you get about the same or better effective benefit.
I love space projects, but there's a Krell-level hubris involved with gigantic projects assuring the theoretical right of every person to smelt aluminum in their backyard.
That's you out there, Morbius.
Thanks, Dr. Murphy. It is starting to become clear that nothing will work. The EROEI for rooftop solar becomes negative with lead-acid battery storage, the best way to store it. Wind is horribly intermittent, requires too many metals, is geographically limited to at most a terawatt worldwide, and requires inefficient backup to the point that greenhouse gas reductions are virtually zero. M. King Hubbert may be proven right that the fission breeder will be the only thing that works, such as the IFR or LFTR. However, we must make the end of growth so obvious and permanent over the next few decades that breeders are never again abused like fossil fuels were.
I live in Oregon now, since it will survive the collapse better than other areas. Are there any other Oregonians reading this who would like to chat about the situation over a cup of coffee?
--Zack
"Who would have thought around 1900 that in fifty years time we would know so much more and understand so much less?"
Albert Einstein
It's not at all clear that 'Nothing will work' .. unless you are suggesting that for it to work it has to keep life just the same as it is right now, or keep providing us Cheap and Effortless Energy Slaves. Many of our own ways have to adjust to be part of making things work as world energy reality shifts .. but that doesn't negate the usefulness of the tools themselves, in any case.
Lead Acid is certainly not the only way to store the power from Solar Electricity, and you didn't show that Solar Heating is unworkable. It's probably one of the best heavy-lifters we've got, since such vast amounts of electricity and fuel are spent just to create 'Low-grade Heat', for Space Heating and Water Heating, cooking and drying. Solar heat can also work to drive refrigeration, too.. and all that heat is quite easily and cheaply stored in a range of materials.
Wind is a fine resource, as evidenced by the great collection of Windfarms going up across the globe.
I really think you've taken away some very unconvincing conclusions from this discussion. (I do tend to think Space-solar is a very unlikely contender, though) Maybe you're looking at them all individually, and not as a combined collection of tools that can each do a bit, and even if the sum total might be a good bit less than we know today.. that's still our opportunity to waste, isn't it? The tools you question are already out there working, across a range of scales and continents..
It's just odd to me that you find all these renewable sources questionable, but are willing to bank your image of our future on Breeders.. that's putting all the eggs explicitly into one, largely unproven basket.
I mentioned this in my earlier reply to Ghung, but will do it again.
I'm no expert on PV, I just put it together myself as an inexpensive hobby project, didn't even read any articles about it since I like figuring out stuff for myself. It seemed to make sense to use most of the power in the daytime, and just store enough for computers & lighting at night. This drastically scales down the number of batteries needed. I installed a low-voltage disconnect to make sure the batteries never cycle too low, so they should last a long time... years now with no degradation I can tell. Inexpensive timers and switches make it possible to do stuff like refrigeration while the sun is shining. For the freezers, I used the low-tech expedient of plastic bottles of salt water which freeze to ice during the daytime. This works fine even at the small scale I'm doing it.
EROEI is a great concept since it embodies a Copernican-level insight: there are absolute limits even for a race of pyromaniac apes. However, we live at a time in which fossil fuel, and in particular oil, are being burned as fast as they can be extracted from the ground. In such a society where we're basically flaring it for dumb reasons (dopamine now in exchange for a wrecked planet in the near future), EROEOW (properly pronounced as a howl of existential self-realization) makes a bit more sense... "energy return on energy otherwise wasted". Human society did not burn more fossil fuel to make the batteries and solar panels for my garage, it simply slightly reallocated the energy used in making different crap. There's an essential fungibility to the making of crap. DJ's Paradox (with apologies to Jevons): Anything that doesn't alter the extraction flow rate of fossil fuels doesn't cost or save energy.
It is true that nothing is likely to keep multiple billions of humans simultaneously alive indefinitely, but that too is an indulgence. The place for billions or even trillions of humans was deep time, not land area. Though we've likely messed up the "trillions" thing, and for no real reason. PV & lead-acid: it's good enough for anything we really need to do, and a whole bunch of what's just convenient or fun to do. No reason not to get some while the flaring continues.
Greenish. Thanks a very lot for making the point I have tried to make so often- "simply slightly reallocated the energy used in making crap."
My point, often expressed to no response whatsoever, is that it is nuts to say, for example, "a switch to solar costs too much" when all we have to do is start reallocating the resources spent on very very obvious crap. We then trade some stuff way far down on the 'needs" hierarchy to get stuff way higher up. How can anybody argue that 'COSTS TOO MUCH"?
And since we have a huge resource of energy, etc, allocated to crap-(70%?), reallocating it could go a very long way toward getting us where people here say they want to go.
Yet I see the "costs too much" thing here over and over , dammit.
I'll respond only to say Amen to that.
Isn't it remarkable how seldom this is brought up by anyone?
Most folks just assume that we need mountains of crap. They assume we need full baseload power 24/7/365. The list of dumb indulgences goes on and on. Of course we have enough energy left to transition, and we could do it while leaving most fossil fuels unburned, with pretty simple tech. But we're a population of tribal-ape minds which are not - in aggregate - sentient or sapient, so the the extent we communicate about such stuff at all we're the captives of delusional narratives about "need", and captive to our highly stimulated hedonic ratchets.
When you get right down to analyzing reasons we might need a geosynch solar armada even if it did work, it mostly comes down to neurotransmitter dysfunction in a race which feels it needs to be going somewhere fast at all times.
I'll stand by my contention that for the most part, fuel is just being flared for no good purpose.
So here's a request from this tired old grandpa to all you energetic young'uns, Please keep this theme going, with lots of examples of crap just waiting to be discarded in favor of not-crap. "I've got a little list---they never would be missed" , with maybe an oft-repeated thread of ever- extended examples-
I toss in for starters- all fuzzy stuffed animals from china, any passenger car with more than 4 cylinders, big fat pickup trucks ( and their big fat drivers, if I had my druthers) lawn mowers and lawns, mediocre restaurants, anything that can't be sold unless advertised, hair lotions, bottled water, trips to the mall on impulse--.
But I'm getting tired just naming them. Carry on.
I'll add a couple needlessly 'flared' fuels..
- Driving an errand you could pretty easily walk. (I think many of us do this)
- Disposables .. anything from ziplock baggies to cell-phones that we replace and dispose of long before their usefulness has expired.
'Did you think you could kill time and not injure eternity?' - Thoreau
The EROEI for rooftop solar becomes negative with lead-acid battery storage, the best way to store it.
Lead Acid batteries are scrap after a few years.
But nickel-iron don't suffer from the scrap after a few years issue of Pb-Acid.
I've got nothing against nickel-iron or any other chemistries, but isn't recycling of lead-acid batteries pretty easy?
Lead is also much more valuable than iron.
What does it cost to buy the new battery and how much do you get for the scrap price?
Just because the dead battery is able to be broken down and mostly remade as a new battery - shouldn't one be looking at things that skip the re-cycle step?
These people are trying to make an "open source" battery:
http://opensourceecology.org/wiki/Nickel-Iron_Battery
Lead Acid lifespan can be a good few years, though, and afterwards, that scrap is reusable.
Did Tom's Battery article do an energy life-cycle for recycling Lead Acid Batts?
I hope that's an industry we can rebuild Stateside, and get it happening with proper EPA controls. (Long Live the EPA.. watch your backs!) I heard that very little of it still exists in the US currently.
I would think that Lead Smelting and Refining would be a heat process that could be supported with Solar Heat, as that goes.. it's pretty low temp to melt.. does it require much more energy at other process stages?
My lead-acids were built near Chattanooga less than two hours from us. The recycling plant is nearby. Electric arc furnaces at both. They also made the batteries on the LA Class sub I was on:
http://www.hawkerpowersource.com/
...and Rolls/Surrette batteries, some of the best, are made just up the road from you Bob:
http://www.rollsbattery.com/content/company-faqs
Great to know.
I'd heard almost all the Lead Recycling had gone to the Far East.. glad the hold-outs are reputable!
Thanks.
Because ERoEI does not become negative, I assume you mean less than 1. Do you have a reference? How much energy is used to manufacture an L-16 lead-acid battery?
This eroei claim is common, and what comparisons I've seen don't account for the energy costs of grid connections, maintenance, coal, natural gas, their extraction costs, etc.. Think of the immense amount of energy involved in placing stuff in GEO, including infrastructure, wasted materials, support staff (and their energy consumption),,,etc. My PV/battery set has been operating for years with only sunlight and water, along with minimal maintenance from me.
I am 93 million miles from my energy source. The stuff I need to utilize it is all within about 30 meters from where I sleep. I like it that way, though this relationship to my energy acquisition and use disqualifies me from participating in these discussions in a way ("forgive them, for they know not what they do.."). It's quite the opposite of "out of site, out of mind", that most of you seem to prefer. While I acknowledge the need for centralized sources of energy to provide for the commons, many of the comments here are indicative of the mindset that got us into the absurd situations we all find ourselves in, the mindset of those who are utterly reliant upon evermore concentrated and complex sources of power and control, all-the-while concentrating the consequences. I prefer to pay my own piper.
I am thinking in terms of my off-grid PV system. I notice the total lifetime energy from my 8, L-16 batteries (7,000 cycles between 90% and 100% charge yields 13,300 kWh with a lifetime of 19 years) is comparable to the energy output by my PV panels over 19 years (15,600 kWh). My batteries cost me $180 each and currently cost $323 each (not including sales tax) which creates a price range of 10.8 cents/kWh to 19.4 cents/kWh of lifetime energy returned which is more than the wholesale price of electricity likely paid by the manufacturer. One can not determine the energy invested from the price, but the price / kWh is consistent with the claim that the ERoEI of a L-16 battery is less than 1. Because the people making this claim declare ERoEI is negative, they do not understand ERoEI, and I suspect the claim is based on the price.
From page 16 of Life Cycle Assessment of Five Batteries for Electric Vehicles Under Different Charging Conditions (Michail Rantik, KFB-Meddelande 1999:28, PDF warning):
Lead-Acid Battery (MJ/kg battery)
My Crown L-16 batteries weigh 54 kg each, so the energy to manufacture one of them is 362 MJ = 101 kWh.
The manufacturing ERoEI of one L-16 battery compared to the lifetime energy discharged from the battery is (1659 kWh) / (101 kWh) = 16.4.
Combining discharge at night and from high power loads, I use about 1 kWh from my batteries per day, the efficiency of the batteries is ~75% over their lifetime, and the PV panels output about 2.25 kWh/day on average after deducting for cloudy days.
Energy returned from batteries = (1 kWh/day * 6939 days) = 6939 kWh
Energy invested to manufacture and charge batteries: (101 kWh * 8 batteries) + ((1 kWh/day)/.75)* 6939 days = 10,100 kWh
Energy output from PV panels = 2.25 kWh/day * 6939 days = 15,600 kWh
If the energy payback time for the rest of my PV system is 3 years, then 2.25 kWh/day * 1096 days = 2466 kWh of embodied energy.
Assuming a 19 year lifetime for batteries and PV, ERoEI of my residential off-grid PV system is at least 15,600 / (2466 + (10,100 - 6939) = 2.8. Because most of my PV panels are over 19 years old, the actual ERoEI is higher than 2.8. Adding more PV panels would also increase the ERoEI. Zachary's statement is wrong.
You can't just park your power station anywhere you like in geostationary orbit.
Wikipedia: Space Law
Space wars on the way.
This was my first objection - I recall many years ago I had heard there were only 160 geosynchronous slots, which is about how many satellites are up there today. Even assuming we can increase that by a factor of 10 it will be tight, and the least crowded spots are over Kiribati.
There is also a wonderful write up (Scientific American?) about how easy it would be to launch a small projectile (marble sized), reverse it's orbit with the moon and send it against traffic (6km/s ΔV) in the anti-geosynchronous orbit. A multi-trillion dollar sabotage do-able by anyone with launch capability, like Lex Luther, the Joker or Goldfinger.
They don’t.
The reason for the slots is the uplink. In order to reuse the uplink frequencies, the satellites have to be far enough apart for the uplink microwave beams not to overlap. There is no reason a communication satellite could not be attached to a power sat. The limit on the number of power sats is eclipsing. But by putting them in slightly angled orbits with the nodal points over noon and midnight, they can be spaced much closer.
I can't decide )given the three fictional fictional characters mentioned) if you are serious or not. A power sat, particularly a thermal one where most of the area is light reflectors, is not going to be noticeably damaged by a 1 cm projectile. Plus the fact that one of the uses of propulsion lasers would be to clean up the space junk, and it's also likely to be used against a loop around the moon rocket.
100 kg of #00 buck shot, about 286,000 pieces, placed in a retrograde orbit at 35,800 km. would give each one of these pieces an energy of about 67,000 J. In about one day there would not be a single operational satellite in GSO, and there is no need to loop around the moon to do this.
Have you considered the size of the rocket you would need for 100 kg payload in retrograde GEO?
I suspect you don't know how to calculate this since you dismiss the loop around the moon.
I, like many here, am a space romanticist turned space realist. The Orbital Solar Power Dream won't be realized anytime soon (or perhaps ever) for the same reason the Colonize the Solar System Dream won't be realized: at the end of the day, anything we can do in space or on the surface of another planet will be harder than the same thing done on Earth.
Even with dramatically lower launch costs, building a self-sufficient, expandable habitat on Mars is just... hard!
Even an Earth ravaged by rapid climate change, comet or asteroid impact, or even nuclear war is an infinitely more workable environment that Mars or the Moon. Earth has a thick, oxygen rich atmosphere, abundant water, and protection from solar and cosmic radiation. If the surface and its resources were contaminated beyond recovery, blasting and digging deep into the crust is still easier than blasting ourselves up into the heavens.
"Even with dramatically lower launch costs, building a self-sufficient, expandable habitat on Mars is just... hard! "
In an environment where 20-200 5 GW power sats were being constructed per year, it's not a particularly hard task.
Start by sending a power sat fitted with propulsion lasers to Mars. It provides all the energy you need for changing velocity on incoming and outgoing ships plus it would be more than enough to power vehicles to and from the Mars surface on water as reaction mass.
I live in Gulf Shores, Alabama, and all this month it has been 50th anniversary of something to do with the space program and Werner Von Braun. With Hunstville and the Space Center being part of the statewide news site. The issue of Dr. Von Braun's Nazi past came up and the V-2 program. One of the commenters pointed out the V-2 killed far more folks building it, than it ever killed with its warhead.
When Fukushima happened, I remember some folks discussing how nuclear is wholly dependant on fossil fuels, especially during construction and for emergencies. I also saw some fairly eye opening data on how a nuclear plant may actually be a net energy loser in the long run when this is all factored in. When I read this particular story, it sounded more promising than the other examples I mentioned, but how many fossil fuels would you use to build this and keep all of this running?
CC
"but how many fossil fuels would you use to build this"
A *lot* to get started, about a tanker of LNG a week to make the hydrogen that is used for the rocket planes.
"and keep all of this running"
Could be zero.
After a couple of years, it would be practical to switch to electrolysis hydrogen. The energy payback time is under two months.
Funny how these sort of extreme technologies will be considered ahead of a far simpler (technically speaking) solution: inviting all the religious leaders and PTB to a talkfest in the Bahamas and laying out the problems associated with sustaining 7, 8, 9 billion hungry human mouths.
We're a bunch of dumb asses. Very sad.
You're right, very sad.
I always feel a little uneasy writing in defense of SSP. It makes me sound like a committed advocate, whereas the reality is more complex. For the record, I think SSP is technically feasible, could be economically attractive, would be environmentally benign, and has a snowball's chance in hell of getting implemented anytime soon. The chain of developments that would lead to it is too long, and there are no business models I can see that would lead naturally to progression along that chain. Not when there are other viable alternatives. (And I believe there are.)
Nonetheless, I hate to see SSP take a bum rap. There were several points in your article, Tom, that struck me as unfair. Starting with "How much better is space?" In casting the analysis in terms of full-sun equivalent hours per day, you're presuming a model in which the dominant cost of a system is area of solar cells. That might once have been true, but it isn't now. The cost of the solar cells themselves is down to something like a third of the overall cost of an installed PV system, and shrinking.
I realize that advocates commonly use the metric of watt-hours per day per cell area to claim a big advantage for SSP. In that light, it's fair for you to point out that it's not that big an advantage. But even at that, I'm dubious about your 3:1 figure. Most studies I've seen have concluded more like 6:1. I can't argue with the NREL study from which you took your numbers, but I have to wonder if those numbers properly account for solar losses at low angles of elevation, and for average panel losses for dust and reflection from the cover glass?
Be that as it may, a key point that you didn't mention is that the full-sun equivalent hours you used for a 2-axis tracking panel apply to an isolated panel. In any real array, the numbers would be lower due to shading by neighbors. To minimize shading and approach the numbers you used, the surface area occupied by the array would need to be around 5x the cell area, or more. That's one of the reason why solar farms that have been built to date haven't found it worthwhile to employ tracking. They can harvest more energy from the same land area at lower cost by using a denser packing of fixed modules.
In my opinion, the two metrics that matter most in comparing space-based and surface-based solar power are availability and land footprint. Space-based scores way above surface-based on both those metrics.
Enough for now. More later, if I have time.
Good points.
The NREL data does account for low angles, based on measurements that by design incorporated real-world effects like low clouds, haze, etc. But dust accumulation is not included.
You are absolutely right that tracking platforms must be spaced out, losing land density. So the 3:1 is indeed a panel/system comparison, rather than a land area thing (which is essentially irrelevant in space anyway). But this is why in comparing the energy onto the equivalent land-area for the antenna, I placed the panels flat on the ground: not even any tilt. In arriving at the 3:1 ratio, I was trying to answer the question: if you used the panel in its optimal configuration on Earth, how much better is space. I was surprised to see just 3:1, and thought that was worth pointing out. But in the end, it's the myriad other practical considerations that prevail—for which SBSP has a longer list of obstacles.
Land area is still relevant for space-based solar, but it's the land area of the rectenna that applies.
If we're talking a 25% sunlight microwave beam intensity and 85% conversion efficiency, we're looking at 5.1 MWh / hectare / day for space-based, vs. 0.5 MWh / hectare / day for ground based solar in the Mojave. The latter assumes E-W rows of optimally inclined panels, with 50% area coverage, 15% PV conversion efficiency, and the 6.6 equivalent hours that the NREL study gave for such inclined panels. The 50% area coverage allows space between rows sufficient to avoid shading of the winter sun, and for cleaning and maintenance access to the panels. I believe that's about the configuration utlities have found most cost-effective for ground-based solar.
Bottom line, 10:1 in favor of space-based on the land use metric. And that's before counting the fact that the rectenna area can be dual-use for agriculture. Add the near 24/7 availability, and you can appreciate why SBSP looks interesting.
But the cheap launch and space-based assembly / maintenance infrastructures are BIG hurdles.
"But the cheap launch and space-based assembly / maintenance infrastructures are BIG hurdles."
Exactly. I don't see a way to get cheap launch that doesn't have one heck of a front end cost. Open to idea on this point. Even if the cost can be repaid in a 2-3 years that a big hurdle to get over.
But if you do have cheap launch, then I think most of the rest of the problems go away. Food and other resupply for 1000 workers is around a 1/3 of a percent of the parts traffic.
I think assembly is going to take a jig a little bigger than a power sat. Rolling sheet metal into beams takes a couple of roll formers run at walking speed. A few spot welders seem to be enough to weld all the joints. The productivity in tons per person per day was a small multiple of making Liberty ships in WW II. I have not put much time into designing power sats, but it seems there are lots of ways to get the job done.
Other than the fact they tend to be far from the factory (on average), a production facility making a GW of power sat every three days should be up to repairing or replacing ones that fail.
Roger,
I think you are off by a factor of 10 for your desert based solar array...
1ha = 10,000 sqm. 50% of land area = 5000 sqm. Panels at 15% = ~150w/sqm
5000 X 150 X 6.6 = 4.95Mwh/hectare/day.
Bottom line, 1:1 on the land use metric.
Er, no. It's still 10:1 in favor of SBSP; I was off by a factor of 10 for both. I must have used 1000 m^2 / ha instead of 10,000.
Land area is still relevant for space-based solar, but it's the land area of the rectenna that applies.
PV can be placed on existing roofs and doesn't necessarily need additional land area. Rectenna's cannot.
In fact over 80% of the PV capacity in Germany is placed on already existing roofs.
Bottom line, PV on the earth-surface requires even less land area.
Rooftop solar could be viewed as "dual use" of collector area for housing, instead of agriculture. But small job installation costs and retail equipment make it 2x - 3x more expensive than utility solar farms. Plus fewer full-sun equivalent hours in most cases, less than optimal orientation, and in many cases, partial shading by trees during the day. Average kWh per m^2 per day will be quite a bit less than the ugly rows of panels in the Mojave.
I'm not knocking rooftop PV, but it's not the most cost-effective option for weighing against space-based.
PV on profiled sheeting roofs is cheaper per W than any ground based installation even if you ignore the costs of additional transmission lines.
Here's offer for a complete 46 kW system for €1.4 /W: http://www.photovoltaikforum.com/angebote-f41/2500-46kwp-1409eur-46kw-%C...
This thinfilm PV desert plant cost $2.94 /W even though it is 1000 times larger:
http://en.wikipedia.org/wiki/Copper_Mountain_Solar_Facility
Also rooftop PV is not exposed to sandstorm, competes at end-user electricity pricing while desert PV competes at wholesale electricity pricing.
Besides: Roof top PV is undoubtedly significantly cheaper than space-based PV and it is undoubtedly much more prevalent than desert based PV. So there's absolutely no reason to ignore roof top PV, even if it was more expensive than desert based PV.
Rooftop solar could be viewed as "dual use" of collector area for housing, instead of agriculture.
So you are basically claiming that the antenna's are essentially invisible and cover 0% area and that having cattle and fruit pickers exposed to this microwave beam among your invisible antenna is essentially the same as having people in this PV covered factory which produces 450% more energy with its PV covered surface area than it requires...

"cattle and fruit pickers exposed to this microwave beam"
Are you concern with ten plus kW/m^2 inside your microwave oven when standing in front of it and warming up your coffee?
Don't mislead, that's contained inside the oven. You aren't exposed to it. Your exposure is many orders of magnitude less.
"Your exposure is many orders of magnitude less."
Exactly. The rectenna backing stops the microwaves from going through just as effectively as the mesh on your microwave door does.
the land area of the rectenna that applies
And this rectenna - is it made of magical fairy dust or actual limited materials that actually cost money?
They cost. The largest single expense is the inverters. You can scale the price from PC power supplies which have a nearly identical parts count.
Antenna, diodes, support poles, I cost it out at $200/kW including the labor to plow in the power collecting cables. $200 M/GW or a billion dollars for a 5 GW plant.
Let's take this number as a benchmark and absurdly assume that there's no wind, no hydro, no biomass, no geothermal, no CSP, no interconnection, no nuclear and no gas power.
California get's possibly about 20% on the worst day compared to what it gets on average: 5 kWh/kWp.
So in order to produce always at least the average a PV system would need 5 times more PV-modules to avoid any storage for more than one night. (It wouldn't need 5 times more inverters just 5 times more PV-modules).
Given the fact that $1 PV-modules can be purchased:
http://www.sunelec.com/
An overbuilt PV system would then possibly cost 4 * ($1_module+$1_extra)/Wp + $3/Wp for the basic PV system including inverter.
Keep in mind: In Germany complete small PV systems are built for less than €1.6 /Wp: http://www.photovoltaikforum.com/angebote-f41/31061-26kwp-1574eur--t7478...
A battery pack costs $500 /kWh: http://www.dailytech.com/Model+S+Wont+Air+Until+Next+Year+But+Tesla+Alre...
http://www.dailytech.com/Tesla+CEO+Calls+Nissans+Leaf+Battery+Tech+Primi...
Assuming every night 2 kWh/kWp storage is required, it would add $1000/kWp or $1/Wp
So you end up with $12 /Wp for a ground based PV system with an absurd 100% capacity factor.
Hi Everyone,
I am a huge space fan, would love to see humans colonize Mars and feel I am a fairly expert on space. And I don't think Solar Power Satellites (SPS) can be made to work.
The people who usually argue for this, suggest setting up a lunar base and mass driver, with tele-operated machines & robots to do most of the grunt work. The solar cells are made on Luna and sent to Earth geo-orbit. The first one would be hugely expensive, but once the system is set up, massive numbers of them would be made cheaply.
However, I don't buy it. Solar cells on the ground are just too many times cheaper. And sooner or later the thing will be damaged or go haywire, and then the repair cost is... well... astronomical. (Actually, I'm being unfair. If there is that much infrastructure in space, repairs would likely be part of the job.) But it still seems too expensive to me.
However, rather than spending many billions of dollars building power lines from the Arizona solar farms, thru many, many 'not in my back yards', to where the power is used, you could use a satellite as a bent horn to direct power beamed up in the desert back down to where it is needed. This sort of utility actually would make some sense - especially if it proves very difficult to get the right of way to build thousands of km of huge power lines. I think that if launch prices drop by even a factor of 10, then a Power Relay Satellite (PRS) could make money.
PRS are discussed in the book "Entering Space" by Robert Zubrin.
Also it is perfectly possible to lower the cost into space by a huge amount. Look up Sky Ramps (there is a very nice website on this subject). Basically you build a railway, pointing at the sky, on an east facing mountain and use a rocket sled (or turbo fan powered sled) to boost your rocket to Mach 2 to 3 before it launches. It is already above most of the air, and the running start gives it a huge advantage, increasing the payload by 25 to 40%. Also, the sled glides back down the rails and can be reloaded within a couple of hours. This makes the lowest stage of the rocket, (which is by far the most expensive stage), 100% reusable, low maintenance and with a super fast turn around time. Combine this with a bid dumb booster and you are approaching $100 / kg to Low Earth Orbit (LEO).
I gave a lecture on Sky Ramps at the last Mars Convention in Dallas. They liked the lecture so much that I was asked to write up my speech for their magazine. They cut it to 1/3 of its length, but (for a time at least) the full article with math was on their website.
Warm regards, Rick.
"I am a huge space fan, would love to see humans colonize Mars and feel I am a fairly expert on space"
If you really want people on Mars, there is nothing like a huge power satellite project to reduce the cost of getting stuff into space. Also, moving a power sat to Mars would provide propulsion to and from Earth and local up and down to Mars surface.
"And I don't think Solar Power Satellites (SPS) can be made to work."
Turn the problem around. What would it take for power sats to work? Start with what price it would take to displace coal.
"bent horn to direct power beamed up in the desert back down to where it is needed"
Sorry to say but the math doesn't seem to work in economic terms for space based microwave power relays. Loses for high voltage transmission are in the 5% range and microwave transmission is around 50. So for up and down, 75% of the power would be lost.
Rick, Thanks for the comment. This whole thread has been fantastic fun (pun?), Reminds me of the great bull sessions in the grad house so many years ago, where no idea, no matter how crazy, was off limits, as long as it didn't violate physics. I do believe we brought up all of the stuff mentioned here, including the sky ramp, the mining the moon, and all that. But we all knew it was nothing but beer-induced baloney- and huge fun.
I am sure, from all above, that many others here had identical experiences. But I keep thinking of the guy who was trying to shave grams off of his racing bike by super-clever tricks of structural design, and when I pointed out that with far less effort, he could shave kilograms off of himself, he got a little huffy.
So here, wouldn't it be far far easier to make big cuts in the energy usage of this hugely wasteful civilization, before doing gymnastics in near earth orbit, no matter how entertaining that might be?
I'm still waiting on a demonstration project that shows wireless energy transfer is even possible for Space based power, given the requirements:
Distance,
Power level,
Duty Cycle,
Reliability,
Weight & size,
No consumables like liquid helium to make antennas superconducting.
And anything close to 50% efficient electricity in to electricity out.
Yes, Keith, "There are problems with space based solar power, but getting the energy down by microwaves is
not one of them." The show stopper."The show stopper."
I presume you are not qualified to have done an actual analysis. That being the case, how about finding a microwave engineer, just one of them, who says there are reasons, even one reason, why microwave transmission of power will not work for GEO to earth surface. I would actually appreciate it if you can find someone qualified with a reason that cannot be easily refuted.
BTW, the same power levels, though usually at somewhat higher frequency are extensively discussed in the 698 page NASA publication that was released about a week ago.
http://ntrs.nasa.gov/archive/nasa/casi.ntrs.nasa.gov/20120002761_2012003...
I have a list of fairly nitpicking objections to this work, but the NASA people doing this study never question that beaming of high levels of power is feasible. In over forty years of study of power satellites, nobody has ever called into question microwave energy transfer.
Try google on the subject.
Err-Page 698 is a blank page, or maybe you linked the wrong publication. This deals with Beamed-Energy Propulsion, which is quite feasible.
ETHER
Not saying microwave power transmission isn't possible. Just that it can't meet the requirements for SBP.
"This deals with Beamed-Energy Propulsion, which is quite feasible. "
How can beamed energy propulsion, which needs GW of laser or microwave energy be feasible and power sats which needs GW of microwaves not be feasible?
"it can't meet the requirements for SBP."
Can you be specific about this? I.e., what have world class microwave experts like Willaim C. Brown missed? http://en.wikipedia.org/wiki/William_C._Brown
I find the requirement to stay on a non moving 10 km target from GEO with a beam steered *exactly* like a phased array radar http://en.wikipedia.org/wiki/Phased_array_radar to be much easier than keeping a laser beam focused on a fast moving vehicle over the same distance with single meter accuracy. The latter is what you need to get the lift cost to GEO down to where power sats make any since economically.
The aiming of the laser is certainly a challenge, but so much else is ignored in you cost calculations (in the 2011 key post here). Maintaining a workforce in orbit will be exponentially more expensive than maintaining oil workers on the North Slope (and oh how the oil companies whine about that). The low launch costs are only achieved once dense launch schedules are in play. The build up to these points, assuming it is possible, will be enormously expensive and cannot be dismissed as insignificant relative to fuel costs once the whole show is up and running. Tens--more probably hundreds--of billions of dollars will evaporate leaving precious little usable residue during the build up phase.
another comment you made above showed a major reality disconnect
Also, if you can't project this into displacing fossil fuels in a decade or so, it may too little, too late
Ten years from now for untested tech to fully functional orbiting human manned manufacturing/assembly/maintenance and laser boost facilities?!?!?!
And you think China with its nonexistent manned space background is going to be the country to accomplish this?!?!?!?
"much else is ignored in you cost calculations"
Of course. I am one person with a few friends helping who tried to capture the major element and left lots of margin for the rest.
"Maintaining a workforce in orbit will be exponentially more expensive than maintaining oil workers on the North Slope"
It's the ratio of what they produce to what they cost that's of concern. Take resupply at 10 kg/person/day. At $100/kg that's $1000 per person per day.
If 500 people are making $500 M of power sats per day, the resupply fraction is one part in 1000. For back of the envelope calculations you can ignore numbers this small.
"Ten years from now for untested tech"
Think Manhattan Project. Is this harder? The energy disaster seems to be about 20 years out. I am assuming that if this every gets done it will take ten years of arguing about it. Power sats have been under consideration since 1968.
"And you think China with its nonexistent manned space background"
The Chinese seem to have more capacity to put people in orbit right now than the US does. They are seriously more motivated. Japan too.
While I would like to see it done, it's also important to show that the energy crisis has at least one reasonable cost solution. There may be other ways that are better.
Think Manhattan Project. Is this harder?
Think of needing aircraft needing resupply in route to deliver the product of the Manhattan project to a distant island and having having only a navy of rowboats and a couple large kites to start out with.
Power sats have been under consideration since 1968.
man had considered powered flight for millennia before the Wright Brother short hop. It will take much faster movement than early aviation had to have this show up and running in a decade. Reflected laser ignited propulsion needed to get orbiting loads to geo is about as fully unproven as things get.
Not to make too light of the physics and tool design involved in stimulating nuclear fission but it is generally a lot harder to build big stuff than to make little stuff that will tear it up. We are having a heck of a time just hitting rockets with other rockets at right now. Mirroring beams tens of thousands of kilometers--it will take more than a few failures-that is after all the setbacks setting up the orbiting facilities.
Its the buildup to the cheap launch, and all the design missteps and unforeseen problems that will have the great cost. Fifty billion dollars will barely be a drop in that bucket, at least that is my opinion having seen construction overruns and pilot project boondoggles on rather banal terrestrial design/build projects for decades.
If 500 people are making $500 M of power sats per day, the resupply fraction is one part in 1000. For back of the envelope calculations you can ignore numbers this small.
It is the complexity of having 500 people trying to do that in the space environment that will be extremely costly--and of course wages per person will be in excess of your given resupply cost, shift rotation will add more. It is tough to keep a large crew healthy to boot--all those insignificant items will add up to a few percentage points of cost not tenths of a percent-which puts even more pressure on the $/kg to geo price, which already needs to be shaved razor thin which apparently must happen during a completely subsidized launch schedule build up phase.
The energy disaster seems to be about 20 years out. I am assuming that if this every gets done it will take ten years of arguing about it.
If something like this is to be attempted, the best guess for full cost from concept to full implementation needs to be out there and then prudent decision makers will multiply that figure by a factor of ten to a hundred or so and maybe have a price number that is a bit closer to the mark.
Of course many would now argue the energy disaster is already here and seven billion strong making further discussion pointless. So carry on and good luck, any effort you make is better than sitting on the rock and crying.
The Rock (1944-1948) Peter Blume
Always stop in front of this for a while when I visit The Art Institute of Chicago
Shift rotation is costly enough to consider just sending up families and leaving them there for years.
Not shaving the cost to GEO, we are taking an ax to it. Takes a different approach to do it at all. The rough cost for shipping things up using lasers comes in at around $37/kg. That's amortizing the rocket plane, hydrogen fuel/reaction mass, refurbishing the rockets and a reasonable write off of the lasers. (Writing off $50 B in 5 years is $10 B/year. The income from shipping 500,000 tons at $100/kg is $50 B.
The project completely fails its goal if the cost of power can't be brought to under 2 cents per kWh. Ten times makes it 20 cents per kWh, we can do better with ground solar including storage. 100 times would make it two dollars a kWh. That's just out of the question.
That's what I figure. :-)
I'm just completely amazed at the amount of handwaving that's done here! That is too expensive? We'll just do this. No consideration at all the the far reaching effects of this. Not to mention the Social Engineering.
"just sending up families and leaving them there for years" Have you considered if you'll get that many families? You know you're not going to get that many to practice abstinence for that time, don't you? Are you also planning supply schooling, childcare and healthcare? You're also going to have to have medical professionals and facilities. Is all this included in your "estimates"? And, no, all your multiple orders of magnitude too optimistic estimates don't have enough padding to cover it.
"The project completely fails its goal if the cost of power can't be brought to under 2 cents per kWh." Realistic cost estimates come in too high? Just declare them too expensive and ignore them.
Any time a reality-based problem is pointed out, the handwaving occurs and the the problem is totally dismissed. Reality? That's for those who can't handle Fantasy! (To paraphrase Lily Tomlin.)
I've always been a fan of space exploration, manned and unmanned, but this reads like a very badly written SF pulp novel, complete with Alien Space Bats.
I've been trying to politely hold back some kind of rant that sounds just like this.
I really don't want to be rude to the people who have clearly worked hard to crunch whatever numbers they have, and feel that this has some semblance of potentiality.. but as you mention the handwaving that accompanies any question about how hard space installations will probably prove to be, when we try to scale them up to 'community' sizes.. eg, how much of a ground-force will be required to hang onto the 'fat end' of an umbilical that supports the away team, similar quite probably to the amount of fuel and money and danger it takes to deliver fuel and other bare essentials to the front lines in Afghanistan.. well, it brings mind to a term I've coined exclusively from my time listening to Oil Drum discussions on this and that..
"Irresponsible Extrapolation" ( probably would be a subchapter in the book on Explosively Expanding Complexities ) .. sometimes the functions go far beyond merely Exponential as they attempt to scale.
I have reason to think larger sizes will be less of a problem than small ones. Some things just work a lot better at large sizes, some, like power sats and microwave don't work at all till they get really huge.
Take a laser powered rocket. It seems not very hard to keep lasers on a 10 meter square (120 ton rocket) with a one meter pointing accuracy. It's nearly impossible to get them to work if the target is only a meter.
A large part of the time of the people on the ISS is taken up in maintenance. If it were expanded to 100 people, the maintenance would increase but not by the larger number of people.
The ground force for keeping the workers fed and clothed isn't going to be a lot larger than the staff it would take to support a store in a community of 500-5000 people. Now they are going to using up parts building power sats at 60 tons per hour, and some of those parts are going to have substantial complexity. The cost of the parts before they are is transported to GEO is ~$180/kg. On the other hand, lots of car factories make more complicated objects at more than 60 tons per hour.
My numbers are all out there, public domain except where I have not yet posted them. If you don't like them suggest where they should be changed.
For example, 10 kg/person per day resupply. Do you find that unreasonable?
What I find unreasonable in your projections, is that we have 6 Human Beings in Space at the moment, according to http://www.howmanypeopleareinspacerightnow.com/ .. Is it possible that we've ever had more than 20 outside of Earth's Atmosphere at once, for more than a couple days' camping trip?.. and then, have really got a handle on this whole range of challenges in creating an environment that even 30 or 40 can not just survive, but actually live and remain healthy in for extensive periods of time?
You could point to Submarines, or to McMurdo, I suppose, but even those have a considerable number of 'Earth Givens' and they have a few Escape and Quick Access options that make their sustenance currently managable.. but the backup of Navies and Aircraft provide at least a few parallel and established avenues to build a reinforced network of mobility and experience that we simply don't have in space yet by a long shot.
It's like expecting Baron von Richthofen to be able to fly an F4 Phantom. The jump from where we are to what you're describing has to be bridged with (IMO) a real foothold in Space, and 6 guys on the truly still Nascent Space Station seems to be a really thin slice of experience and infrastructure from which to make the leap you describe. And it will take THAT experience to really see what we need out there, where the next string of surprises and complexities are going to come at us from. The idea of cost projections and suggesting feasability when we're this inexperienced is what makes this read as a 'nice wish', but not really realistic.
And at this point, as we're already possibly flatlining on Oil, I don't see where the margins or buffer will be coming from to create such a wonder. That spare energy is going to be thin, and we've got to develop a new transportation infrastructure, insulate houses, redesign cities and Ocean Fleets.
regards,
I don't especially care about this debate - space solar just seems like an unnecessary distraction.
Still, I gotta quibble: the US, at least, has an enormous reserve of energy consumption that is used for really, really marginal values: single occupancy SUVs as the best example. The US could get along just fine on 50% as much energy.
We have a huge margin.
But how would that excess ever be made available for a use like this? I don't think it could, so I still don't see an argument for how that makes this any more feasible.. which is to say that I basically am just detailing why I agree with your first statement there.
The new consumer would outbid some current users.
If diesel were needed, they'd bid what was needed to induce a percentage of truckers to put aerodynamic hoods on their cabs, or shift to NG; and/or push a certain percentage of freight customers to rail.
That price rise in diesel would induce a few refiners to shift their mix from gasoline to diesel, raising gasoline prices. Gas prices would rise just enough to induce a few Parents to take the Corolla back from the Teenager for the daily commute, and force the teenager to drive the SUV on dates (as far as possible on savings from summer jobs).
Eventually, of course, Teenager would nag Parents into buying a Prius/Volt/Leaf and giving back the Corolla, and life would be good again...
Mass of the space station is about 390 tons. Rated at 6 people or 65 tones per person. Because of the design it's not very efficient. Bigelow Aerospace says at the 100 person size, 5 tons per person is plenty even for a rotating habitat. At the design materials flow rate of 60 tons per hour, the ISS, which took most of a decade to bring up would be brought up in 6 1/2 hours. A 5000 ton habitat would come up in 3.5 days.
This is just a consequence of the material flow rate required to make a dent in the energy and carbon problems. Nothing new, nothing faster, just bigger.
The main energy input to this project is LNG. "LNG imports into Japan alone this year are expected to hit a record of 79 million metric tons." Ramped up to full capacity, the LNG consumption is about twice the hydrogen consumption of 76 tons per flight x 3 flights per hour, 460 tons per hour x 80,000 hours per year. or about 36.5 M tons per year. That's about 1/2th of the LNG Japan will use this year.
It's a lot of LNG http://seekingalpha.com/instablog/365869-keith-schaefer/375851-the-lng-s... Typically LNG tankers are around 200,000 cubic meters or 143 tons making the consumption around 5 ships a week. US consumption is about 426 M tons per year so it would be about 8.5% of US consumption or around 2% of world production. Of course within a year of starting to use gas at this rate, 100 GW of coal or natural gas fired electric generators could be shut down.
If I have the numbers right, does this look like a good investment to you?
"If I have the numbers right, does this look like a good investment to you?"
I don't believe theres ANY way to have the 'numbers' right at this point.
We didn't build the Space-shuttle by making Modifications to tbe B-17. I said very clearly above, we need relevant experience and some support infrastructure in place.
We will not be putting dozens of people out there if we have such poor capacity for getting to them and helping when wierd stuff crops up. I can't believe it. And that's just one angle on how this is all far too 'thin' to work at this point.
"Good investment" was in reference to the amount of energy in the form of LNG this project would take and the number of power sats produced per year from that energy input.
"we need relevant experience and some support infrastructure in place"
Of course. Gaining that experience is part of the project.
"dozens of people out there if we have such poor capacity for getting to them and helping when wierd stuff crops up."
If production is going, the delay to get there is single digit hours. The minimum energy Holmann transfer orbit is 5 hours and the headway between loads is 20 minutes.
The worst part comes when there are only a few up in the early days of constructing the propulsion laser at GEO and Falcon Heavy flights are in the one a week to one every other day range. That's still a lot better than the current situation with the ISS.
If we decide to do it before the ISS is dumped in the ocean, my suggestion would be to use ion engines to push the ISS up to GEO and use it as a "construction shack."
Unlike astronauts, who are not expected to die (even though lots do), construction workers are *expected* to die in the course of large projects. If this really concerns you, hire it out to the Chinese. Accidents could kill the whole construction crew 6 times over every year and not come up to the Chinese death rate from coal mining. Developing energy is dangerous, as the 11 killed in the Deepwater Horizon explosion shows.
Should be no problem. The Mohawk iron workers and their families moved from Canada to NYC in substantially larger numbers. The L5 Society before it merged into the National Space Society had 10,000 members who were itching to go into space. We need ~500 for the 100 GW level, 5000 for the 1 TW level, and if we are serious about putting carbon back n the ground, at least 10,000 for a two TW/year production level.
Don't be silly. There is birth control, but for something that's going to continue for decades, why not plan for a relatively normal community?
Of course.
Certainly
Yes.
Wait a minute. I estimated the number of workers based on the WW II production of Liberty ships and a small multiple for 70 years increase in productivity per worker. Much of the machinery would be automated, the workers are there mostly to unjam the machines when they screw up. (And set them up in the first place.)
And now much of a multiple do you need for the medical professionals and teachers? Ten percent seems reasonable, it's certainly less than a factor of two, not "multiple orders of magnitude." Look up the numbers for a remote mining operations.
Not at all. If "Realistic cost estimates come in too high" the project makes no sense economically and we have to find another way to cope with the energy and carbon problems. The point that originally surprised me was that the resupply for keeping a large workforce in GEO took a very small fraction of the transport to build power satellites at a rate where they could contribute to solving the problems.
Well, this isn't exploration, it's more like a construction job or a remote mining camp in the 1800s. And I did write some SF that included a mess of iron workers building power sats. It's part of the same sequence as "the clinic seed" but not completed or published. I took it through to an asteroid mining camp where the children of the Mohawk iron workers are about to graduate from high school. If you want to see it, ask.
[Deleted] Forget it...
So how does a giant orbital mirror (at geosynch) compare cost-wise? Build the ground panels, run them on sunlight while you put the mirror in place. Once the mirror is functional, you get power 24 hours a day (plus bonus power from direct sunlight).
The mirror should be possible to build paper-thin. Mylar with some reinforcing bands or something similar. If your panels can handle the additional output you can keep adding more mirrors later.
It's not technically feasible. The problem would be control. The mirror would need to be on the order of 10 km in diameter, and the surface would need to be aimed and maintained with sub-milimeter precision at all points in order to reflect the beam to its target location. With a passive mirror, there's no way to get around the pointing problem. Can't use trick of phase conjugation of a pilot beam, the way an active transmitter would.
That's a lot of constant light and heat for the neighbors to have to buy into..
On a planet that already has warming concerns due to the energy from the Sun being trapped in the atmosphere.
If global warming is taken as a fact, how does taking more photons from the Sun and injecting them into the biosphere is a good plan?
It surely isn't. I just thought I'd point at what would kill the plan first, and having a 24/7 beam of multiple megawatts of concentrated solar hitting nearby would probably make for a pretty displeasing new star in the sky, and a big beam visible for possibly many hundreds of miles. Didn't think it needed much more comment.
If a bit more sunlight is offset by burning less coal for power, how much net warmth are you adding? And global warming isn't "taken as a fact" anyway, since the globe stopped warming in 1997.
The correct solution for power needs is advanced fission plants (gen4 Thorium, etc), but I'd vote for orbital generation just to get the space program boosted in the process. Lowering cost to orbit is key to getting our heavy industry moved into orbit.
In 2020 space based solar power will be half the cost of nuclear power
Entech expects that by 2020 their panels will generate 1KW per kg. That means a 1 GW solar panel would weigh 1,000 tons. We could launch that into LEO for $2.6 B using Falcon-9 (then use ion drive) .... half the cost of an equivalent nuclear power station.